A new source of X-ray fluorescence for art
Author: Álvaro Peralta Conde is a senior researcher at CLPU (Pulsed Lasers Centre)
Although unfortunately it takes place in rare situations, the synergies between scientific and artistic disciplines offer a vast number of possible applications. The benefits are clear: on one hand artists, conservators and restorers profit from the detailed knowledge about matter and its natural transformation processes that Science provides, and on the other hand, scientists learn to appreciate the immaterial essence of artistic beauty. One of these common places, where all these professionals work side by side, is provided by the technique known as X-ray fluorescence (XRF).
XRF is a non-destructive technique –which is mandatory when working with invaluable artworks- that allows knowing in detail the atomic composition of a sample, e.g., a pigment or a welding. For example, if we irradiate a certain pigment of a painting with X-rays or high-energy electrons, vacancies are produced in the inner shell of the pigment atoms. Other electrons lying in higher energy states rapidly occupy these vacancies, radiating in form of X-rays their excess of energy. Since the electronic structure is a characteristic, a fingerprint, of each atom, by analysing the emitted radiation, it is possible to determine the atomic composition of the sample.
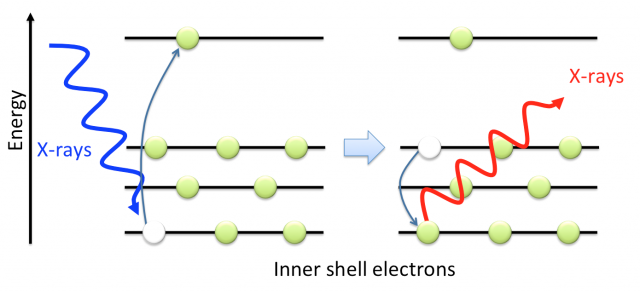
Nowadays the importance of XRF is beyond doubt 1. When applied to paintings, it help the restorers not only to prevent the natural degradation of pigments –for example it is remarkable the work done to avoid the degradation of Van Gogh paintings in the University of Antwerp -, but also to answer questions related to authenticity and provenance of a piece of art. We must notice that the Master painters have their own palette of colours being unique in colours as well as in pigments composition.
![Figure 2. Vincent Van Gogh (1853-1890) [left], Edvard Munch (1863-1944) [centre], and Henri Matisse (1869-1954) [right] palettes. Photographs by Matthias Schaller.](https://mappingignorance.org/app/uploads/2016/11/x2-640x310.png)
However the interpretation of the XRF data is not always straightforward. X-ray emission from the instrument itself and/or the surroundings of the artwork under analysis, e.g., the holder, can induce misinterpretation. Also we must bear in mind the penetration depth of X-rays in oil pigments. Assuming a typical X-ray excitation energy of 100 keV, this depth goes to around 12 cm meaning that the fluorescence X-ray signal is produced not only by the superficial layer (the visible layer), but also by all the layers of the painting. For example, white lead (2PbCO3·Pb(OH)2) was routinely used to prepare the canvas before the painting and hence Pb signal appears as a very strong background signal that may mask weaker ones.
In a recent publication 2 we propose an alternative that may overcome the described difficulties. The key idea is to use a laser-based X-ray and electron source for XRF analysis 3. While X-rays has a large penetration depth, for electrons it is much limited. For example 60 keV electrons penetrates only 50 m in oil paintings. Thus, using electrons as excitation source we can obtain the fluorescence signal of just the superficial layer of the sample simplifying considerably the analysis of the results.
Figure 3 shows a scheme of the experimental setup. For the production of X-rays and electron bunches, we focalize a high-energy femtosecond laser by a microscope objective into a copper target reaching intensities of the order of 1017 Wcm-2 at the focal spot. In simple terms, the generation process can be understood as follows: since the laser intensity is considerably high, even at the early stages of the laser pulse, matter gets rapidly ionized. Once the target is ionized, the rest of the incoming laser pulse interacts with an expanding plasma from the target surface. In this interaction, the electrons –driven by the oscillatory electric field of the laser– are extracted from this plasma, accelerated, and reinjected again into the bulk, producing both, Bremsstrahlung (X-ray) radiation by the sudden loss of energy of the re-injected electrons, and a distinctive X-ray emission spectrum characteristic of the material chosen as target (typically a metal). However, the electric field of the laser does not re-inject all electrons into the bulk, producing the simultaneous and collinear emission of electron bunches and X-rays pulses in the direction of the reflected laser beam from the target. This source of electrons and X-rays can be used jointly or separately by using a magnet that deflects the electrons from the X-ray beam.
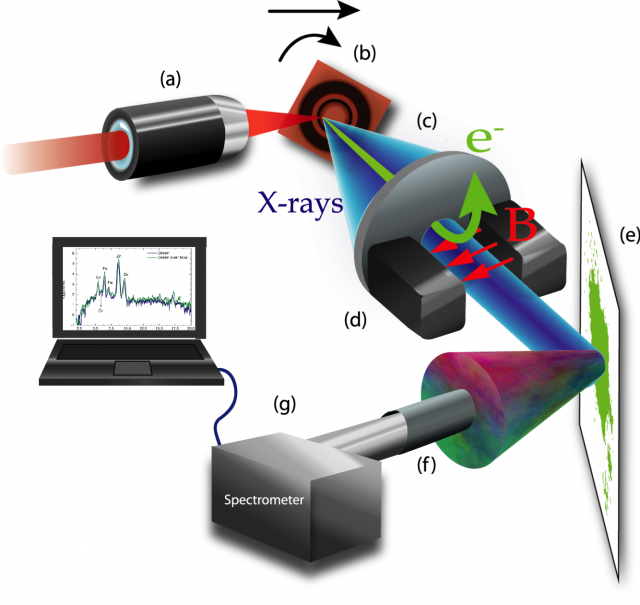
collimator, d magnets, e sample, f collimator, g spectrometer
Figure 4 shows the XRF spectra of a green pigment –emerald green Cu(CH3COO)2· 3Cu(AsO2)2– alone and over a blue pigment –cerulean blue CoO·nSnO2– when excited by X-rays and electrons. In both cases the pigments were deposited over watercolour paper. When X-rays is used as excitation source, we can clearly see the Ti emission lines from the blue pigment (see Fig. 4 right side) even if the blue is beneath the green. Titanium is used in the pigment industry in the form of titanium dioxide (TiO2) usually known as titanium white. However if electrons are used as excitation source on the combined set of pigments, the Ti signal disappears meaning that just the superficial pigment layer (the green one) is excited. Thus, combining both excitation sources, we can have access to weak superficial signals that otherwise would be difficult to distinguish from the background.
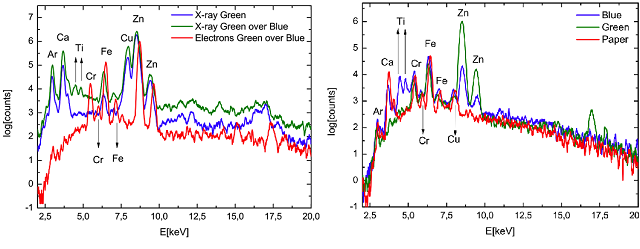
It is important to notice that this application has become possible by the incessant development of laser technology. Nowadays, lasers with shorter pulses, more energy per pulse, and more friendly to operate are routinely developed being difficult to envision a limit for the technology. This progression has paved the way to new and unexpected applications, some of them inconceivable just a decade ago. In this work, lasers advances helped to bring a little bit closer Art and Science, and maybe to convince professionals of both fields of the benefits of interdisciplinar collaborations.
References
- Handheld XRF for Art and Archaeology. Studies and Archeological Science Series. Leuven University Press, 2013. ↩
- Laser based X ray and electron source for X ray fluorescence studies, F. Valle Brozas, A. Crego, L. Roso, and A. Peralta Conde, Appl. Phys. B 122:220, 2016. Arxiv: https://arxiv.org/abs/1605.04075. ↩
- F. Valle Brozas, A.V. Carpentier, C. Salgado, J.I. Apiñaniz, M. Rico, M. Sánchez Albaneda, J.M. Álvarez, A. Peralta Conde, L. Roso, Rev. Esp. Fis. 29, 3, 17-21, 2015. ↩