The Higgs boson, the No-Lose theorem and the W(boson)ardrobe
We are almost sure: we have discovered the Higgs boson. Or at least, that is the most reasonable comment to do after the usual Winter Report given by the scientists that work on the two main experiments placed at the ring of the Large Hadron Collider (CERN, Switzerland), named CMS and ATLAS, the last December 13th.
However, during the last years there was a big controversy about wether the Higgs boson existed or not that even caused large doubts to the europeans, as well as to their politicians, about if it was reasonable to spend such a huge amount of money (the cost of the accelerator was estimated up to 2010 to be of 7.5 billion euros) in an experiment to look for a particle that couldn’t be there. However, physicists didn’t share those thoughts and doubts not because they wanted to build the LHC and all the detectors at any cost but because they had great reasons to know that there should be something there whether it was the Higgs boson or not.
The story starts with the completion of the socalled GWS model (Glashow, Weinberg and Salam) that accounts for the unification of the electromagnetic and weak interactions in the electroweak one in the first years of the 70’s, together with the discovery of the W boson in 1983 at the SPPS collider at CERN (one of the secondary accelerators that are used nowadays to inject protons into the LHC) and was generated by a huge problem of the theoretical model when studying interactions, in which two W bosons collide, something happens and another two W bosons are produced at the end of the process.
Such kind of interactions are produced in the GWS model via three possible diagrams (three possible interchanges of particles between the initial and the final states) that account for the cross section of the scattering, which naively can be understood as the probability of having such interaction when colliding two W’s. Those diagrams are interchanges of Z bosons and photons, which can be interpreted as if the two W’s produce such particle, that later decays again to two W’s; and an elastic collision between the two bosons provided by a 4-leg vertex. The problem is that the full cross section of the interaction, computed as the sum of the cross sections of all the individual processes, grows monotonically and very strongly with energy, reaching a point, below the TeV scale, where it produces probabilities greater than one, pointing out that something is wrong with the model.
The solution is introduced in the Standard Model, the model that we use nowadays to describe not only the electroweak interactions (consequently it includes the GWS model) but also the strong one; by adding the Higgs sector to the matter content there is another possibility of a process that contributes to the scattering, which is the interchange of a Higgs boson between both pairs of W’s. This new contribution to the cross section of the full process is such that the divergent part of the total computation that was responsible for pushing probabilities over one is suppressed, so the total result is coherent with all well known laws of physics.
However, until this year we didn’t know if the Higgs boson actually existed (we still do not know it for sure but uncertainties are getting smaller every day) so physicists didn’t know if that was the real mechanism to solve the problem but, for sure, there had to be something to solve it under energies of 1TeV, so Higgs boson or not, building the LHC was not a whim. This idea, that the accelerator should be able to see something, whatever that statement meant, is called by theorists “No-lose Theorem” and nowadays there are versions of it 1 for the next accelerators that will come after the LHC to search for new proposed particles.
Moreover, this statement about the hard scattering is very important since it can be used as an independent test to check if the new particle found by ATLAS and CMS is or is not the famous scalar boson with scottish surname so, of course, both experiments at the LHC have measured the cross section of this process looking for possible deviations of the Standard Model that could make us hide the theory in the bottom of a wardrobe and look for another one.
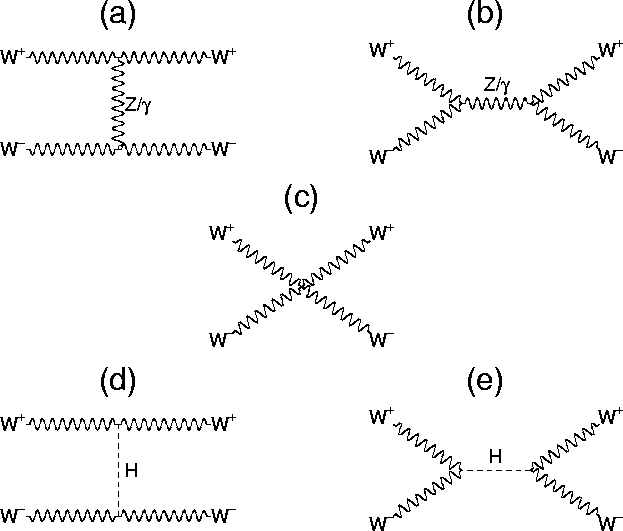

However, looking at this cross section and finding the Higgs influence is not easy. As computed by CERN theorists 2, the enhancement of the production of final states above the one due to all the other possible processes that contribute to
production at the energy at which the LHC operates is in the best case of a barely 5%, to which we have to add the fact that what detectors see are not the W’s but its decay products. That is the reason why the data analysis is so important in particle physics and why it takes far more time than the collisions itself since the physicists have to decide, by kinematical arguments about how the momentum of the outgoing particles should be, what collisions are dependable enough to be considered without doubt as coming from a
process. That is why particle physics is a slow but trustable path. And I say trustable because due to the huge amount of statistical data needed in order to obtain an experimental result, the conclusions of a high energy experiment are very accurate and they can be trusted in ensuing experiments, like in the case of
, in which the extensive experience of physicists after SPPS, LEP and TeVatron experiments is used to reduce the uncertainty about the distribution of the decay products of the W boson.
As you may expect, the ending of this story is a happy one. Once the two experiments detected a possible signal for a new particle, it was possible to compute theoretically the rate of bosons produced due to a Higgs boson of such mass and to compare data with theory. That is one of the things that has been done using all the data collected by CMS and ATLAS during these last three years of operation, examining all the collisions searching for
signals and asking whether they behave as the ones predicted by the Standard Model 3 or they do not. And boy, as the figure shows, they do.
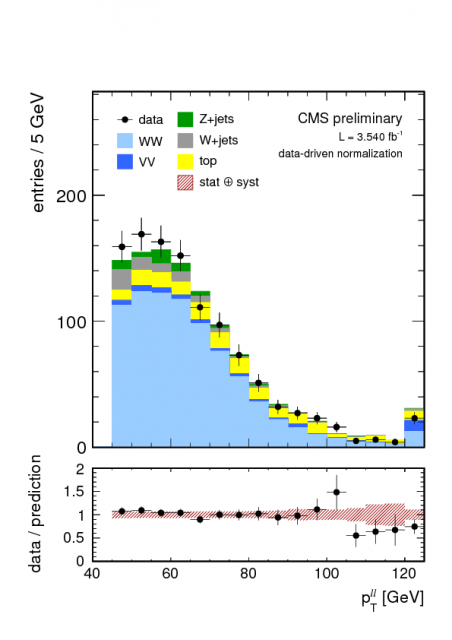
References
- José R. Espinosa & John F. Gunion, No-Lose Theorem for Higgs Boson Searches at a Future Linear Collider Phys. Rev. Lett. 82, 1084–1087 (1999) DOI: 10.1103/PhysRevLett.82.1084 ↩
- John M. Campbell, R. Keith Ellis, Ciaran Williams, Vector boson pair production at the LHC, Journal of High Energy Physics July 2011, 2011:18 DOI: 10.1007/JHEP07(2011)018 ↩
- CMS Physics Analysis Summaries, Measurement of WW production rate, Report number CMS-PAS-SMP-12-013 ↩
1 comment
[…] Matt Strassler…“Todavia, existem tantos modelos e parâmetros, que não há ilusão de teoria perfeita – apenas uma fortuita […]