Hot Saturn and the thermal history of the giant planets
All of the giant planets of our Solar System, except Uranus, emit more energy in the long wavelengths than what they receive from the Sun in the short wavelengths. This excess of energy was generated in the early stages of the planetary formation and it is being radiated slowly to space as the planets keep shrinking and cooling. In principle, it is possible to know from thermodynamics how the planets will reduce their luminosity as a function of time. For doing so, we need to assume some simplifying hypothesis on their structure and thermal behavior. It is very common to accept that they are homogeneous bodies and that their atmospheres behave adiabatically, not exchanging heat with their surroundings. Not surprisingly, neither of these assumptions hold completely, and their behavior significantly departs from what one could expect. The good news is that understanding the observed deviations of the planets from idealization leads us to a deeper knowledge on how the planets were formed and on how their atmospheres behave today.
Since the 70s, we know that Saturn is too luminous in the thermal infrared. This conundrum was initially explained by the complex life of Helium in Saturn’s interior. The idea was that, being smaller, Saturn cooled faster than Jupiter and eventually the Helium in its interior condensed and rained out. This would produce an additional source of energy and this should create the excess of energy we are seeing. Most recent studies, however, show that it is not possible to explain the observations based solely on Helium rain.
In this context, a recent paper by Jérémy Leconte and Gilles Chabrier in Nature Geoscience 1 elegantly shows a possible explanation for Saturn’s thermal excess. What they propose is a mechanism termed “layered convection” which is usually neglected but that could be the key ingredient for cooking a hot Saturn. A vertical gradient in composition is able to stop the large-scale convection that is commonly assumed for the giant planets interiors. Basically, this is what we can find in Earth’s ocean. Instead of having a single convective cell from the top to the bottom, we have a number of small convective cells separated by some interfaces in which diffusion is dominant over convection and corresponding to discontinuities in the composition of the atmosphere.
Leconte and Chabrier argue that planetary conditions lead to a state of layered convection and develop and simple semi-analytical model for the thermal evolution of Saturn. As we can see in Figure 2, atmospheres with layered convection are less eager to cool down and so they retain information on their initial state for a longer time than the pure adiabatic atmospheres. At early stages, an adiabatic planet would be more luminous than a planet with layered convection but at a certain point of its evolution the one with layered convection would start to be brighter. This model is able to explain at the same time the current luminosity of Saturn as well as other constraints such as the planetary radius or gravitational moments.
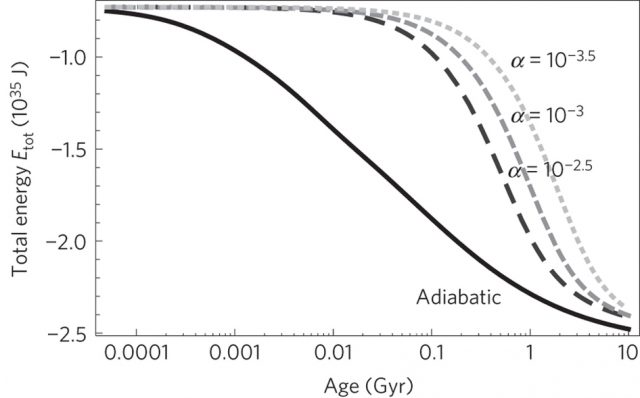
The exact way in which the planet is releasing the heat from the interior plays a central role in its atmospheric dynamics. During the Voyager mission in the early 80s, it was possible to measure Saturn’s emitted power in the infrared wavelengths. It was found to be symmetrical and pretty constant in latitude, while the incoming sunlight is not. As the tilt of Saturn’s rotation axis is similar to that of the Earth, the planet’s equator receives far more light than the poles. This implies that something is redistributing energy throughout the atmosphere and this probably feeds the active meteorology we can see in the giant planets.
The observations by the Cassini spacecraft orbiting Saturn since 2004 made things even more interesting. In a paper by Liming Li and collaborators 2, it was shown that the apparent symmetry in Saturn’s thermal emission was broken (see Figure 3), possibly by the role that cloud cover plays in modulating the energy release. So it becomes apparent that there is a link between the thermal evolution of the giant planets and the current meteorology and cloud cover we can observe. We just have a glimpse of this complex link, but it is clear that all the elements of the problems are interrelated.

Could these ideas be applied to other planets, different from Saturn? Leconte and Chabrier argue that the current luminosity of Jupiter, which is in fact lower than one would expect from adiabatic evolution, could be in agreement with the layered convection mechanism stopping at sometime in the past, possibly after the erosion of Jupiter’s core (which has not been detected up to date) or just its soluble components. This would imply that different thermal histories ended up with two very different planetary interiors. Even the missing energy source in Uranus’ interior could have a place in this layered convection scenario. While Saturn has already entered into the time in which it is brighter than an adiabatic planet, Uranus is possibly still being darker than it should and, given the properties of the planet, we possibly need more time to see the planet glowing in the thermal wavelengths as Saturn does. In our quest of extrasolar planets, we have already found numerous examples of thermal properties that are waiting to be explained, so a model like the one by Leconte and Chabrier appears like a substantial contribution to understanding giant planets evolution.