Communication breakdown: schizophrenia as the outcome of subtle neuronal dysfunction
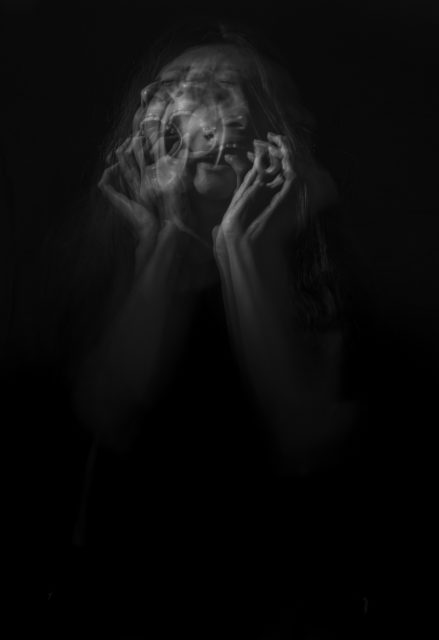
The functioning of the human brain goes far beyond a bunch of cells packed together; each of them is able to produce, receive, and transform electrical signals into a concrete response that affects the neighboring cells, counted by hundreds. This image is already complex, but if we say that the electric signals are constituted by only a fistful of chemical compounds, it gets really puzzling. Moreover, when we realize that the result of this chemical communication between cells can convert different wavelengths into complex images, vibrations of the air into classical music, sequences of symbols into literature; it makes us aware of the world surrounding us, but also permits us to invent whole new worlds.
The human brain is much more complex than we initially thought. The development of biology and its constant feedback with other disciplines such as chemistry, physics, and informatics keeps providing more and more data. Today, it is slightly easier to assume that the chemical dance inside our heads is complex enough as to explain all of the classical attributions to human mind and soul. However, we are far from completely understanding most of the mechanisms converting electricity into poems and sculptures. After many years of descriptive analysis and gross dissection of the most general and easily observable treats of human brain, neuroscience is just starting to be. And when it gets to trying to understand and fix the malfunctioning of human nervous system, we sadly must assume that it is far from being a complete and consolidated field of knowledge.
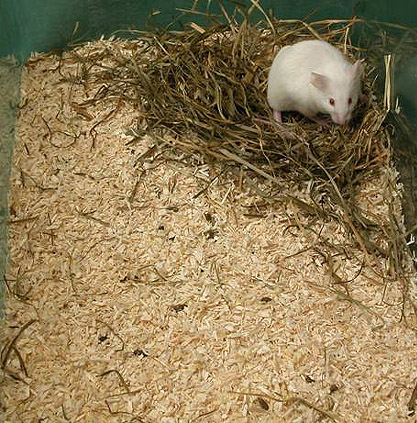
Severe mental disorders are among the most frightening and discouraging pathologies. A healthy body controlled by a sick brain is something we are not prepared to easily handle. We are what we think we are, and we take others as we see, hear, and feel them. But if some error in our brain starts making confuse what we see, hear or feel, our world becomes messy, unstable and threatening. That is why one of the most terrifying diseases one can face is schizophrenia. This terrible pathology comprehends much more than complex auditory hallucinations (popularized, in a very unfortunate way, by many films); it also affects strongly to physical performance, emotional response and social capacities. Schizophrenia is as difficult to treat as to diagnose, since it develops in very different forms and affects patients in a very broad range of symptoms. Although some genetic conditioners for schizophrenia have been described, there is not a clear genetic heritable pattern, and the strong effect of environmental factors as well as the influence of life habits make it even more difficult to prevent; only postmortem analysis, and lately novel techniques that permit to visualize the electrical pattern of brain activity 1, have revealed some common treats in schizophrenia that may lead the path for medical treatment in upcoming years.
In the meanwhile, current research in biomedicine develops smart tricks that permit the deconstruction of the events leading to the neurological abnormalities observed in schizophrenia. There is yet no other way to approach the study of neuronal functions than dissecting piece by piece very complex networks, and reducing, in a simplistic but necessary way, the role of neurons as switches that either activate or inactivate certain circuits. This can be addressed by knowing the particular neurotransmitter (the small molecules that constitute the electric language of neurons) that each kind of neuron produces and/or responds to. In summary, researchers pursue the knowledge of what kind of chemical short-circuits make neurons to produce such complex signals as to be taken by real words.
It is the case of the work by Isabel del Pino and collaborators, recently published in Neuron 2. The starting point is a genetic conditioner for schizophrenia, the protein named ErbB4. This protein acts as a receptor for another molecule called neuregulin, with a very important role in the development of the brain, and which has also been related to the apparition of schizophrenia. Neuregulin binds ErbB4 and permits neurons to correctly migrate and interconnect to each other. Defects in this process have been related with the altered function of a concrete type of neurons, the ones that respond to a neurotransmitter called GABA. Since alterations in GABA response have also been linked to schizophrenia, the goal of the approach was set to determine the implication of the failure in ErbB4 and the mechanism underlying its linkage to development of schizophrenia. Using genetic engineering techniques, the presence of the protein ErbB4 was selectively deleted, in a very precise moment of the development and only in a particular type of neurons, in a strain of experimental mice. This refined genetic alteration (which affected only a type of cells called interneurons, involved in the connection between several parts of the developing brain) permits to study the loss of the protein in a very narrow circumstance, thus avoiding the global and dramatic effects of eliminating the function of a gene with such an important role for the whole brain activity.
An interesting outcome of this alteration was that the overall structure and architecture of the cell types and positions in the brains of genetically altered mice was perfectly similar to that of wild type animals. In other words: they switched off one controller inside the cells, but they did not remove or change any cell population at all. This provided a useful tool to assess the effect of the disruption in chemical signals between particular groups of neurons. What they observed was that ErbB4-deprived cells displayed altered excitatory patterns, and also, could not respond properly to the signals received from other connecting neurons. Besides, the distribution of axons (the long protuberances along which neurotransmitters travel from one end of the neuron to the opposite) and synapses (connections between different neurons) appeared significantly altered. Following the path of the nervous impulses in this particular circuit, it was found that the destination of the connections from this altered interneurons, a type of neurons called pyramidal cells, received a reduced number of inhibitory stimuli. As a result, they were constantly overexcited. To sum up an exhaustive and ellaborated analysis of electrical behavior and cellular features, we could say that the effect of ErbB4 conditional deletion produced a communication breakdown, a confusion of the normal signals that resulted in a global and uncontrolled enhancement in neuronal activity.
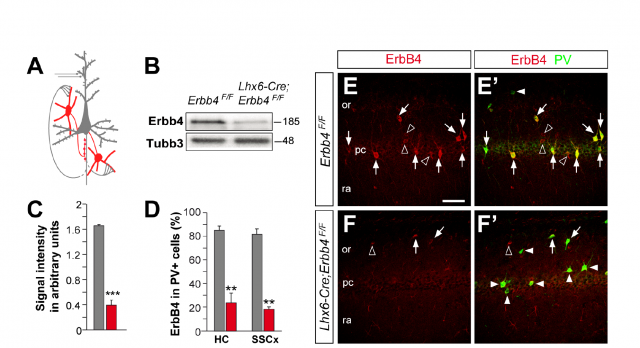
But, what has all this to do with schizophrenia? One of the neuronal definitions of this pathology relies in an altered pattern of neuronal activity that results in overexcitatory stimuli in certain cell populations. These alterations are thought to be the base of the delusionary issues of the disease (usually referred to as positive symptoms). However, it is difficult to ask a mouse about what is going on inside its head. In these cases, there are a series of behavioral tests that permit to understand if the alterations produced in the animal’s brain are affecting its perception of the world: capacity to explore new surroundings, interaction with other mice… the ability to build and maintain a nest, for example, gives interesting clues about organized behavior, planning and working memory. In almost all experiments performed, genetically altered mice showed an abnormal behavioral pattern that can be compared to the emotional deficits in patients with schizophrenia. Although, for sure, there is a long road form a mouse that exhibits a weird behavior, and the complex mental disorder diagnosed as schizophrenia, the correlation between a genetic marker for the latter, neuronal activity deregulation and aberrant behavior, points to ErbB4 and interneurons as key determinants for the development of schizophrenia.
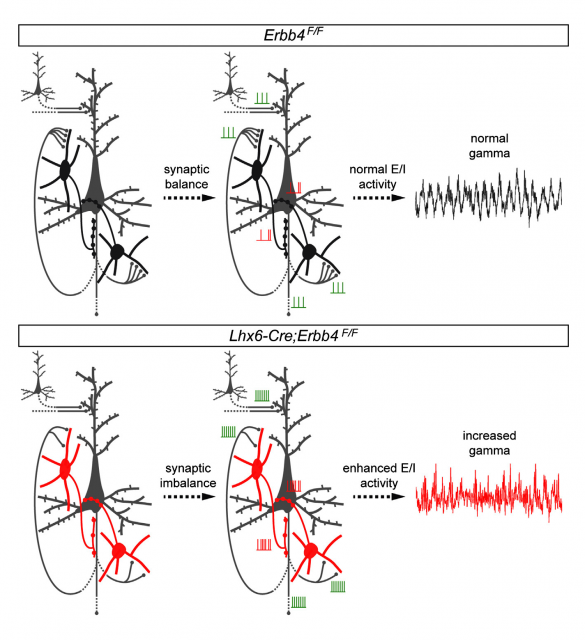
One of the important lessons from this work is the fact that such subtle changes in neuron connectivity can produce a chain reaction that results in a tremendous alteration of brain activity, affecting several cognitive processes related not only to processing information but also to managing memory and emotions. This notion corroborates the strong difficulties in standardizing treatments and reminds us that the patient with schizophrenia holds a devastating battle trying to understand what is going on inside and outside their minds, a battle which we may contribute to withstand only if we take into account such difficulties. The effect of classical pharmacological treatments is extremely heterogeneous, ineffective in a high number of cases, although very successful in others. Neuronal communication requires a critical balance of signals, and this fragile equilibrium lies at the base of a plethora of pathological states which are the result of connecting genetic conditioning, environmental issues and the particular plasticity of the brain of each individual. Only a deep knowledge of each and every signal, and the relationships among cell populations, may lead to the development of strategies based upon concrete molecular targets: maybe we are not so far from discovering the way to turn those switches on and off, at the right time and place, restoring communication between neurons and, hopefully, giving back the patients the control of their lives.
References
- Escudero J., Ifeachor E., Fernández A., López-Ibor J.J. & Hornero R. (2013). Changes in the MEG background activity in patients with positive symptoms of schizophrenia: spectral analysis and impact of age, Physiological Measurement, 34 (2) 265-279. DOI: 10.1088/0967-3334/34/2/265 ↩
- del Pino I., García-Frigola C., Dehorter N., Brotons-Mas J., Alvarez-Salvado E., Martínez de Lagrán M., Ciceri G., Gabaldón M., Moratal D. & Dierssen M. & (2013). Erbb4 Deletion from Fast-Spiking Interneurons Causes Schizophrenia-like Phenotypes, Neuron, 79 (6) 1152-1168. DOI: 10.1016/j.neuron.2013.07.010 ↩