Actinoid oxides, where quantum methods are key to understanding the chemistry
In the standard representation of the periodic table, we find below two separated rows of elements. Their chemistry and electronic configuration belong to a group three element, so what these two separated rows really mean is that in this representation of the table they are all located on top lanthanum and actinium, three-dimensionally building a tower towards the viewer. From an electronic point of view, this is because the d-orbital configuration is shared with group 3 elements, but f-orbitals are being filled.
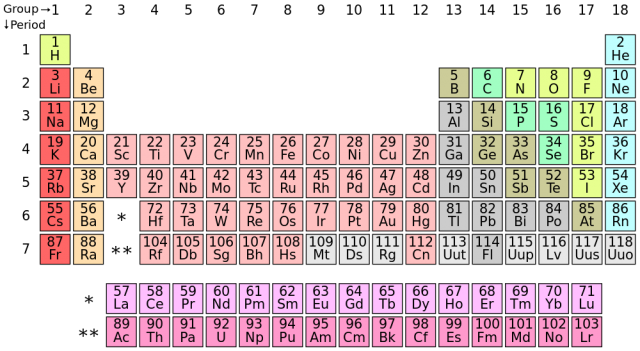
As we have just said, there are two series of metals: according to what IUPAC recommends, the lanthanoids (the 14 elements that follow lanthanum in the periodic table) and the actinoids (the 14 elements following actinium). The lanthanoids and actinoids are collectively known as the inner transition metals, while scandium, yttrium, lanthanum and the lanthanoids are together called the rare earth metals. Although lanthanum and actinium are strictly group 3 metals, the chemical similarity of lanthanum to the elements from cerium to lutetium, and of actinium from thorium to lawrencium, means that lanthanum is commonly classified with the lanthanoids and actinium with the actinoids.
The lanthanoids resemble each other much more closely than do the members of a row of d-block metals, but the chemistry of the actinoids is more complicated.
Actinoids are the heaviest chemical elements with practical relevance. Among them, only thorium and uranium can be found in nature in substantial quantities while natural plutonium has been detected in trace amounts. In uranium ores the radioactive decay of uranium produces transient amounts of actinium and protactinium, while in transmutation reactions isotopes of neptunium, americium, curium, berkelium, and californium can also be formed.1 actinoids heavier than californium are purely synthetic elements, which are made from neutron bombardment of lighter elements.
Along with thorium and uranium, the transuranium elements, neptunium, plutonium, americium, and curium, have important applications and are synthesized in appreciable quantities in nuclear reactors. The other actinoids are mostly used only for research, and the required quantities are produced either in nuclear reactors or in particle accelerators. Neptunium and curium are also significant in nuclear fuel cycles such that their chemical properties need to be understood.
Actinoids most frequently occur as solid oxides, which are the best characterized among actinoid compounds but with significant gaps in understanding of their properties. While solid actinoid oxides, particularly those of thorium, uranium, and plutonium, are relatively well characterized, considerably less information is available on the gas-phase properties of actinoid oxides. The main reasons for this knowledge gap are the large costs and special experimental setups required, such as for high evaporation temperature or laser ablation sources, and the extreme safety conditions necessary to handle radioactive materials, which is a particularly problematic consideration for the actinoids other than thorium and uranium. In addition, experiments are often complicated by the complex vapor compositions and several accessible oxidation states of the actinoids, as well as by a very high reactivity of atomic actinoids with oxygen and moisture.
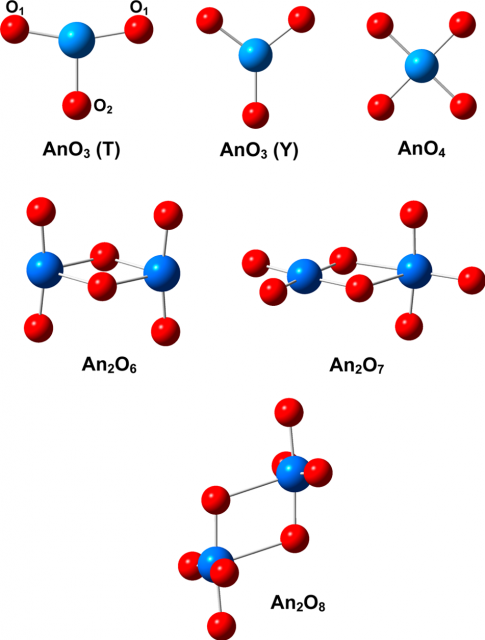
Computational modeling is particularly useful for systems that are not easy to study experimentally, as is the case for most of the actinoids. Quantum chemistry can model molecular properties and transformations, and, in combination with experiment, it can lead to an improved understanding of species containing actinoids. The recent development of theories able to treat systems with a high density of electronic states arising from degeneracy, or near degeneracy, of orbitals, and also with relativistic effects, has led to a rapid increase in the application and advancement of theoretical studies of actinoids. As a result, a substantial amount of theoretical information is available for actinoid oxides, the most ubiquitous and important category of actinoid species.
But all these information available is not of the same quality nor have the same relevance. This is why a group of top actinoid scholars, that includes Ivan Infante from the Kimika Facultatea (UPV/EHU) and DIPC, has teamed up to study1 all the literature regarding molecular actinoid oxides in order to sort it out and establish the trends for several calculated molecular properties assessed by comparison with experimental data results.
The researchers, as it may be expected, find that there are a lot of experimental results lacking. But most interestingly they warn about considering results from state-of-the-art multireference calculations as superior for predicting all properties of actinoid compounds; in some cases more standard density functional theory approaches are way better.
The authors stress the important role of advanced quantum chemical calculations in actinoid research. They are very helpful in both interpreting complex experimental results and for predicting molecular data not available from experiments. The progress in computational science will hopefully soon be able to provide more accurate data and the study of larger molecules at adequate theoretical levels, whereas new experimental studies planned on the less common and highly radioactive early actinoids will provide a broader basis for benchmarking computations.
Author: César Tomé López is a science writer and the editor of Mapping Ignorance.
References
- Attila Kovács, Rudy J. M. Konings, John K. Gibson,Ivan Infante, and Laura Gagliardi (2015) Quantum Chemical Calculations and Experimental Investigations of Molecular Actinide Oxides Chemical Reviews DOI: 10.1021/cr500426s ↩
1 comment
[…] Actinoid oxides, where quantum methods are key to understanding the chemistry […]