Extreme decoherence in chaotic quantum systems
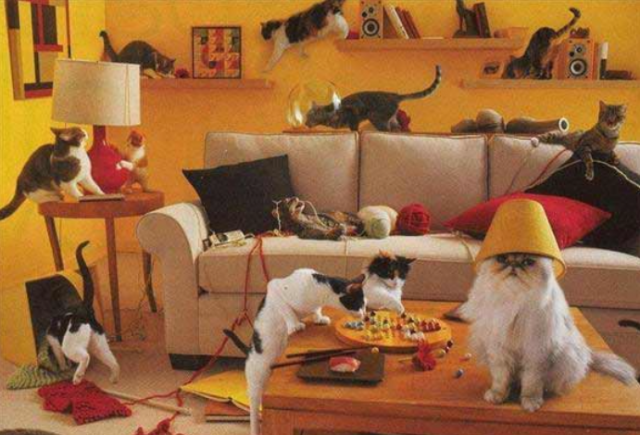
In physics there are some well-known fictional characters: the experimenters Alice & Bob, Maxwell’s and Laplace’s demons, or some astronauts travelling at incredible speeds, to name a few. But the queen of them all is a cat. Yes, you guess right, that is Schrödinger’s cat.
This cat first appeared in a thought experiment designed by Erwin Schrödinger in 1935 with the aim of proving quantum mechanics absurd. The experiment illustrates the paradox regarding the probability of finding, say, a subatomic particle at a specific point of space. According to Niels Bohr’s interpretation of quantum mechanics, the position of such a particle remains indeterminate until it has been observed. Schrödinger postulated a sealed vessel containing a live cat and a device triggered by a quantum event, such as the radioactive decay of a nucleus. If the quantum event occurs, cyanide is released and the cat dies; if the event does not occur the cat lives. Schrödinger argued that Bohr’s interpretation of events in quantum mechanics means that the cat could only be said to be alive or dead when the vessel has been opened and the situation inside it had been observed. Schrödinger’s point was to connect up the oddness of the micro-level to macro-level events.
But, is it really a paradox? Let’s assume that what counts as a quantum entity is any object of any size, like a cat. Then any object could be in a superposition of states, as Schrödinger says and finds absurd. And that would be correct, unless we take a broad view of what counts as a measurement. So, although almost any object can in principle exist in a superposition of states, measurements generally suffice to collapse such superpositions well before we or any other creatures could experience them. Thus, the photon detector used to measure the radioactive decay, the cat herself and so on count as quantum entities that could in principle exist in a superposition of states. However the photon detector suffices to collapse the wave function well before a superposition of cat-alive / cat-dead states could occur. This is what is called decoherence.
In general, decoherence is any process in which a quantum mechanical state of a system is altered by the interaction between the system and its environment. It follows that decoherence must be a ubiquitous phenomenon in nature, responsible for the emergence of classical behaviour from the quantum substrate. Thanks to decoherence Schrödinger’s cat is a paradox no more.
But the implications of the concept of decoherence are much deeper. For example, decoherence is most commonly attributed to the interaction between the system and something external to the quantum system, its surrounding environment. However, it can also arise from something internal, like the presence of random fluctuations in the system evolution. These can have an intrinsic quantum origin, as in the case of continuously monitored systems, or be associated with classical sources of noise.
Noise. If we follow the logic, we will find that the dynamics in each case becomes stochastic, and upon averaging over realizations of the noise processes, decoherence manifests itself in an ensemble perspective, the same concept of ensemble proposed by Josiah Willard Gibbs to talk about the statistical mechanics of thermodynamic systems. Hence, in general, decoherence increases with the system size. This means a real challenge the realizations of quantum information and simulation tasks with complex quantum systems involving a large number of particles and degrees of freedom .
What if the quantum system is so complex that it is chaotic? In this cases decoherence can be expected to be singular due to the enhanced sensitivity to initial conditions.
Understanding the interplay between quantum chaos and decoherence is a long-standing problem. Earlier studies of this subject are mainly focused on the effect of dissipation and decoherence on level statistics, and how to incorporate such effects into the study of quantum systems that are chaotic in the classical limit. Now, a team of researchers, including Ikerbasque Research Professor Adolfo del Campo and Research Fellow Aurélia Chenu (DIPC), poses 1 the question as to what is the ultimate limit to the rate of decoherence of complex quantum systems. This issue is not only of relevance to fundamental and applied aspects of quantum science and technology, but has implications that extend to other fields, including black-hole physics.
In order to find an answer, the researchers introduce a decoherence rate that applies to arbitrary random processes in which the rate of change of a time-dependent quantity depends on the instantaneous value of the quantity but not on its previous history, the so-called Markoffian processes.
Chaotic quantum systems can be described using random matrix theory, originally introduced by Eugene Wigner to deal with the statistics of the spectra of heavy atomic nuclei. Using this theory and the decoherence rate, the researchers show that the dynamics of fluctuating chaotic quantum systems is extreme, in that its rate scales exponentially with the number of particles n. Such scaling has no match in non-chaotic physical systems, where the decoherence rate scales polynomially with n.
These findings suggest that chaotic quantum systems provide an ideal test bed to explore deviations from quantum mechanics, such as those predicted by spontaneous wave function collapse models.
Author: César Tomé López is a science writer and the editor of Mapping Ignorance
References
- Zhenyu Xu, Luis Pedro García-Pintos, Aurélia Chenu, and Adolfo del Campo (2019) Extreme Decoherence and Quantum Chaos Physical Review Letters doi: 10.1103/PhysRevLett.122.014103 ↩