Defeating spin decoherence
Major technological revolutions have occurred when the humankind has been able to harness natural resources, such as fire, electricity or nuclear energy. We are now in the verge of the so called second quantum revolution, that aims to harness two of the weirdest natural resources, coherence and entanglement. This is a tall order that calls for a great dose of ingenuity, because keeping quantum states in coherent superpositions that could be used towards our advantage requires to defeat a rather powerful enemy, the infamous decoherence. Now, Ikerbasque researcher Fernando Delgado (UPV/EHU, CFM, DIPC) and Joaquín Fernánez-Rossier (INL) review 1 the phenomenon of spin decoherence in the context of magnetic atoms deposited on surfaces.
A quantum-mechanical coherent state of a system is that which most closely resembles the corresponding classical state of the system. Thus, decoherence is any process in which the coherent state is altered by the interaction between the system and its environment.
The interaction of quantum spins with their environment introduces relaxation and decoherence in the otherwise fully coherent evolution of ideal closed quantum systems. Spin relaxation and decoherence play a central role in many branches of physics. In the case of nuclear spins, the time scales associated to energy relaxation and decoherence provide a very meaningful information of the environment that forms the basis of magnetic resonance imaging techniques. The spin relaxation and decoherence time scales set the limit of sensitivity in several existing magnetometry techniques, such as optically detected magnetic resonance (ODMR) and spin-exchange relaxation-free (SERF) atomic magnetometry, and are also one of the major constraints in the implementation of spin-based quantum computers, such as donors in silicon, electrons in quantum dots and even molecular magnets.
Moreover, decoherence plays a prominent role in the modern interpretation of the foundations of quantum mechanics, the quantum measurement problem and the quantum to classical transition. In the more specific context of magnetism, decoherence accounts for the emergence of the classical behaviour.
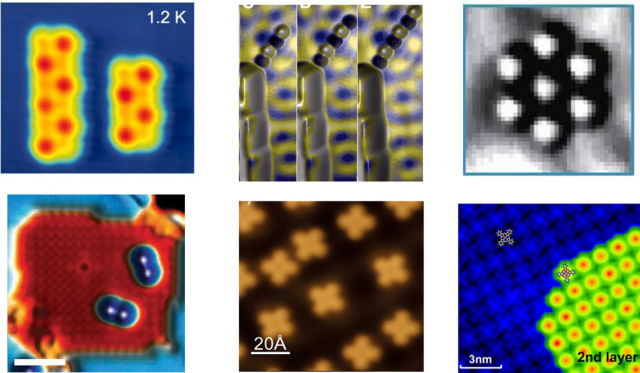
The extraordinary series of experimental breakthroughs in the manipulation (see Fig. 1) and probe of magnetic atoms,mostly using scanning tunnelling microscopes (STM) and in some instances X ray magnetic circular dichroism (XMCD), have allowed the study of the crossover from the quantum to the classical regime in nanoengineered spin structures as well the exploration of coherent dynamics of individual magnetic atoms of surfaces.
The authors goal is to provide a guide for future exploration of spin coherence at the atomic scale. To do so, they try to promote cross fertilization between the traditional STM/atomic scale magnetism community on one side, with other fields where coherent single/few spin physics has been successfully explored using different instrumental techniques, most notably, optically detected magnetometry using nitrogen-vacancy centers, and silicon qubits. The review is, thus, divided in four main blocks.
The first one is devoted to provide a general theory background that yields a proper definition of coherence/decoherence and how to compute it. Particular attention is given to two level systems, given that in many instances one will deal with systems with either a doubly degenerate ground state, such as halfinteger spin magnetic atoms, or systems where the two lowest energy states are well separated from the higher energy excited states, such as spin chains with strong uniaxial anisotropy.
Then, in the second block they illustrate the analysis of decoherence due to the main source in magnetic adatoms (adsorbed atoms), the Kondo exchange coupling with the substrate electrons.
In the third block thay discuss other decoherence mechanism for magnetic adatoms, including spin–phonon coupling and Zeeman coupling to the random environmental magnetic field created by Johnson–Nyquist noise and shot noise.
Finally, in the fourth block, they briefly review the state of the art experimental status to measure spin dynamics with STM and comment on the main challenges to observe Rabi oscillations at the single-atom spin level.
The authors conclude that the exploration of the spin coherence of surface spins by means of STM is just starting but has a promising future ahead. The development of the field will depend crucially on the capability to increase the relaxation and coherence times of the surface spins.
Author: César Tomé López is a science writer and the editor of Mapping Ignorance.
References
- F. Delgado & J. Fernández-Rossier (2017) Spin decoherence of magnetic atoms on surfaces Progress in Surface Science doi: 10.1016/j.progsurf.2016.12.001 ↩
1 comment
[…] Estamos a punto de comenzar la segunda revolución cuántica. En cuanto derrotemos a su archienemigo: la decoherencia. El estado de la cuestión en lo que atañe al espín por el DIPC en Defeating spin decoherence. […]