First nondestructive enantioselective detection technique
If a nucleus has a nonzero spin, it behaves as a small magnet. Therefore, in an external magnetic field, the nuclear magnetic moment vector precesses about the field direction but only certain otientations are allowed by quantum rules. Thus, for hydrogen (spin 1/2) there are two possible states in the presence of a field, each with a slightly different energy. Nuclear magnetic resonance (NMR) is the absorption of radiation at a photon energy equal to the difference between these levels, causing a transition from a lower to a higher energy state.
The main application of NMR is as a technique for chemical analysis and structure determination, known as NMR spectroscopy. It depends on the fact that the electrons in a molecule shield the nucleus to some extent from the field, causing different atoms to absorb at slightly different frequencies (or at slightly different fields for a fixed frequency).
If molecules do not rotate, if they are part of a crystal, for example, some things become possible with NMR. Thus, since this technique provides key structural information, its use for the structural elucidation of proteins, organic, and inorganic materials has become ubiquitous, to the point of becoming an alternative to X-ray diffraction to characterize crystal solids. Actually, the refinement is such that solid-state NMR spectroscopy has been introduced as a real alternative for the analysis and determination of compounds with isotopic natural abundance. Now, if NMR spectroscopy is able to differentiate isotopes, could it be used to differentiate minimum nuances in structure, like those of enantiomers, molecules which are identical except for the fact that one is the mirror image of the other?
Solid-state NMR spectroscopy has two important limitations, though. One is its poor sensitivity for nuclei with low natural abundance such as 15N or 13C and the other the long relaxation times – the average time a system remain in the higher energy state befores it falls to the lower energy one – for these nuclei, which create experimental difficulties. One of the most important techniques to overcome these shortcomings, known as “cross-polarization”, is based on the transfer of nuclear spin polarization from abundant, usually 1H, to less abundant nuclei. This methodology not only enhances the signal strength of the rare nuclear spins but also allows the experiment to be repeated at larger rates because it is governed by the relatively short longitudinal relaxation time Tl of 1H.
Some authors have proposed the use of CP-MAS (cross-polarization magic angle spinning) NMR, in the presence of chiral solvating agents, for determining the enantiomeric excess (ee) of chiral mixtures. It is worth emphasizing that in the absence of a chiral environment the expectation was that CP-MAS would not be capable of generating an enantioselective signal.
Now, through systematic studies of CP-MAS NMR in a series of amino acids, a team of researchers from UPV/EHU, DIPC and ASU has found 1 a rather unexpected behaviour in the intensity pattern of optical isomers in hydrogen/nitrogen nuclear polarization transfer that would allow the use of CP NMR as a nondestructive enantioselective detection technique.
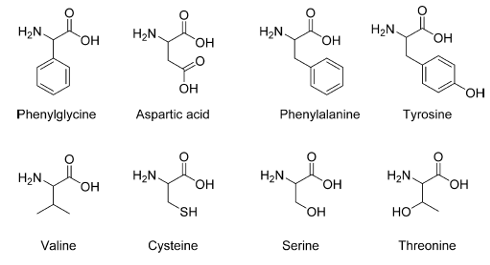
The researchers tested 15N CP-MAS NMR with pure crystalline samples of aeight essential amino acids (Figure 1). Recall that all amino acids, except glycine, are found in two optical isomeric forms, L and D. During these tests, they found a rather unexpected systematic asymmetry in the spectra of the two optical isomeric forms for each amino acid. The intensity for the D form was consistently higher than that of the L form. This differs from all conventional NMR studies of crystalline enantiomeric mixtures. This unexpected finding, which cannot be attributed to other differences in molecular structures, begged an explanation.
The authors attribute this striking result to the onset of electron spin polarization, accompanying bond charge polarization through a chiral center, a secondary mechanism for polarization transfer that is triggered only in the CP experimental setup. Electron spin polarization is due to the chiral-induced spin selectivity (CISS) effect, which would create an enantioselective response, analogous to the one involved in molecular recognition and enantiospecific separation with achiral magnetic substrates. This polarization influences the molecular magnetic environment, modifying the longitudinal relaxation time Tl of 1H, and ultimately provoking the observed asymmetry in the enantiomeric response.
The results are so unexpected and the explanation so new that the scientific community did not remain indifferent and there is some discussion going on. After all, this is the discovery of, to the best of our knowledge, the first nondestructive enantioselective detection technique. The fact that the intensity for the D form was consistently higher than that of the L form, as we mentioned above, is a crucial point to understand what is happening.
If you leave it aside, like Venkatesh et al. did in a comment 2 to this work, we are before what essentially are random effects controlling the relaxation time of the abundant nuclei and the CISS effect should be disregarded. But it is not possible to reconcile the random variation justification with the very systematic result that the intensity of the D -isomer was larger than the intensity of the L- isomer in all cases considered. The researchers address these and other questions in a response 3 to Venkatesh et al. (2019).
These findings challenge some widely accepted ideas about how to use NMR-based techniques to obtain enantiospecific response of pure materials. The authors are currently conducting experiments to further validate their theoretical model.
Author: César Tomé López is a science writer and the editor of Mapping Ignorance
References
- Jose I. Santos Iván Rivilla, Fernando P. Cossío, Jon M. Matxain, Marek Grzelczak, Shobeir K. S. Mazinani, Jesus M. Ugalde, Vladimiro Mujica (2019) Chirality-Induced Electron Spin Polarization and Enantiospecific Response in Solid-State Cross-Polarization Nuclear Magnetic Resonance ACS Nano doi: 10.1021/acsnano.8b06467 ↩
- Amrit Venkatesh, Anuradha V. Wijesekara, Luke A. O’Dell, Aaron J. Rossini (2019) Comment on “Chirality-Induced Electron Spin Polarization and Enantiospecific Response in Solid-State Cross-Polarization Nuclear Magnetic Resonance” ACS Nano doi: 10.1021/acsnano.9b00946 ↩
- Jose I. Santos, Iván Rivilla, Fernando P. Cossío, F. Javier García-García, Jon M. Matxain, Marek Grzeliczak, Shobeir K. S. Mazinani, Jesus M. Ugalde, Vladimiro Mujica (2019) Reply to “Comment on ‘Chirality-Induced Electron Spin Polarization and Enantiospecific Response in Solid-State Cross-Polarization Nuclear Magnetic Resonance’” ACS Nano doi: 10.1021/acsnano.9b00946 ↩