Enantioselective polymerization of a biodegradable polymer using a substituted aminoacid as a catalyst
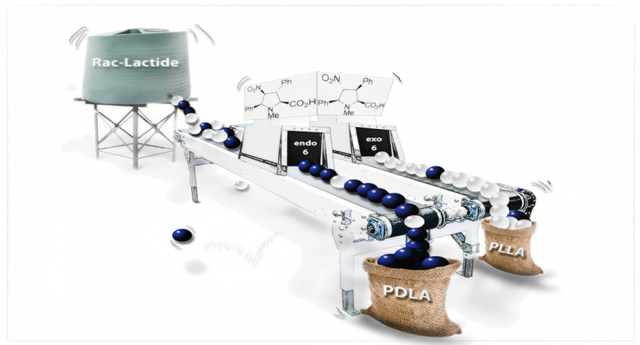
The idea that certain natural products such as rubber are composed of giant molecules, or polymers, consisting of many repeating units linked by covalent bonds arose largely from the work of the German chemist Hermann Staudinger (1881–1965) in the early 1920s. He convinced skeptical chemists of this idea partly by linking small organic molecules (monomers) one at a time through addition reactions to produce larger and larger molecules. His work ushered in an era of systematic study of polymers. It would be difficult to overemphasize the importance of synthetic polymers to present day technology.
Since the discovery of synthetic polymers, there has been a continuous search for control over the different aspects of polymerization, such as kinetics, dispersity, sequence distribution, functionality, and macromolecular architecture.
A special case is when monomers are enantiomers, or optical isomers. Such isomers are mirror images. Any molecule having one tetrahedral carbon atom bonded to four different groups of atoms exhibits optical isomerism. The features of a polymer would vary according to the order these enantiomers present in the macromolecule: just one isomer, or the two of them alternating regularly, or the two of them varying randomly, for example. Stereocontrol, or controling how isomers participate in the polymer, is very important in polymerization reactions because the stereoregularity is a necessary condition for crystalline polymers, which often have an array of mechanical properties superior to those of the corresponding non-stereoregular polymers.
The use of catalysts is quite common in polymerization reactions. Given the excellent performance and almost limitless opportunities of ligand/metal combinations, it is not surprising that transition metals and organometallic catalysts have dominated the field of stereocontrolled polymerization. Stereocontrolled polymerization can be performed by two different mechanisms: chain end control and enantiomorphic site control. In the first mechanism, the chirality of the propagating chain end bound to the catalyst regulates the chirality of the next monomer insertion, while in the latter the chirality of the catalyst, and not the chain end, dictates the next monomeric unit insertion.
One of the polymer families where stereocontrol is attracting great interest is the family of polyesters,especially poly(lactic acid) also kown as polylactide (PLA), as it is made from the cyclic di-ester of the acid. PLA is nowadays the most popular example of a renewable, biocompatible, and biodegradable polymer. Interestingly, the mechanical properties and biodegradability of PLA are linked to its microstructure. Thus, the polymer made exclusively from the L-lactide enantiomer, poly(L-lactide), has a melting point close to 160 °C, meaning it is quite stable, whereas the atactic one (random combination of enantiomers), made from racemic lactide (racemic meaning mix of enantiomers), is an amorphous polymer with low mechanical properties.
Due to the toxicity of conventional organometallic catalysts able of producing stereoregular PLA, purely and safer organic catalysts are desired. Precisely, the search for an organic catalyst family that is able to dictate the monomer insertion (L-lactide or D-lactide from a racemic mixture) as a function of the chirality of the catalyst is an active area of investigation. But to achieve this should not be that difficult: Nature does it continuously. Enzymes are a relevant example, and recently it has been shown that some of them can be reduced to minimalistic analogues such as natural monomeric amino acids like proline without losing the essentials of the catalytic activity.
Now, a team of researchers coordinated by the Ikerbasque researcher David Mecerreyes and DIPC’s Fernando Cossio present 1 an organic catalyst that shows the ability to transfer its chirality to the polymer and to promote stereocontrolled ring-opening polymerization (ROP) of lactide.
Lactide is, as we mentioned above, a cyclic di-ester. And ROP is a form of chain-growth polymerization, in which the terminal end of a polymer chain acts as a reactive center where further cyclic monomers can react by opening its ring system and form a longer polymer chain.
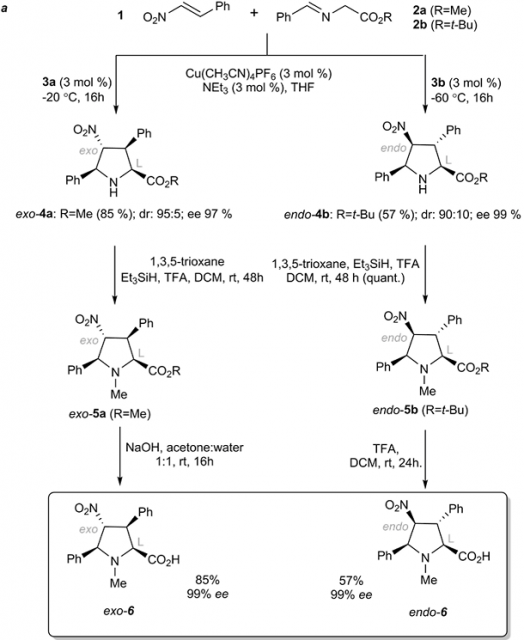
The researchers prepared substituted prolines by means of catalytic asymmetric cycloadditions and subsequent transformations, resulting in unnatural endo- and exo-N-methylated prolines 6 (see figure 2). Then investigated how modifying the chirality of two distal centers of these prolines could promote high stereoselectivity in the polymerization of D-lactide or L-lactide starting from a racemic mixture. Density functional theory (DFT) calculations were also conducted to provide mechanistic insight into the ROP process in the presence of these densely substituted amino acids.
They found that by switching from the endo to the exo configuration in proline 6, the chirality of the catalyst is transferred efficiently to the polymer, thus obtaining a different stereochemical outcome. Hence, when endo-6 was used, it preferentially promoted the polymerization of D-lactide, whereas exo-6 preferentially polymerized L-lactide from a racemic mixture of L and D stereoisomers. DFT calculations revealed the importance of the configuration of the densely substituted amino acids to promote the stereocontrolled polymerization.
This strategy of using combined enantiopure unnatural organocatalyst/organic base pairs could be readily extended to other stereocontrolled metal-free polymerization processes.
Author: César Tomé López is a science writer and the editor of Mapping Ignorance.
References
- Ana Sanchez-Sanchez, Ivan Rivilla, Maddalen Agirre, Andere Basterretxea, Agustin Etxeberria, Antonio Veloso, Haritz Sardon,, David Mecerreyes, and Fernando P. Cossío (2017) Enantioselective Ring-Opening Polymerization of rac-Lactide Dictated by Densely Substituted Amino Acids JACS doi: 10.1021/jacs.6b13080 ↩