Exploring new physics at the European Spallation Source using neutrinos
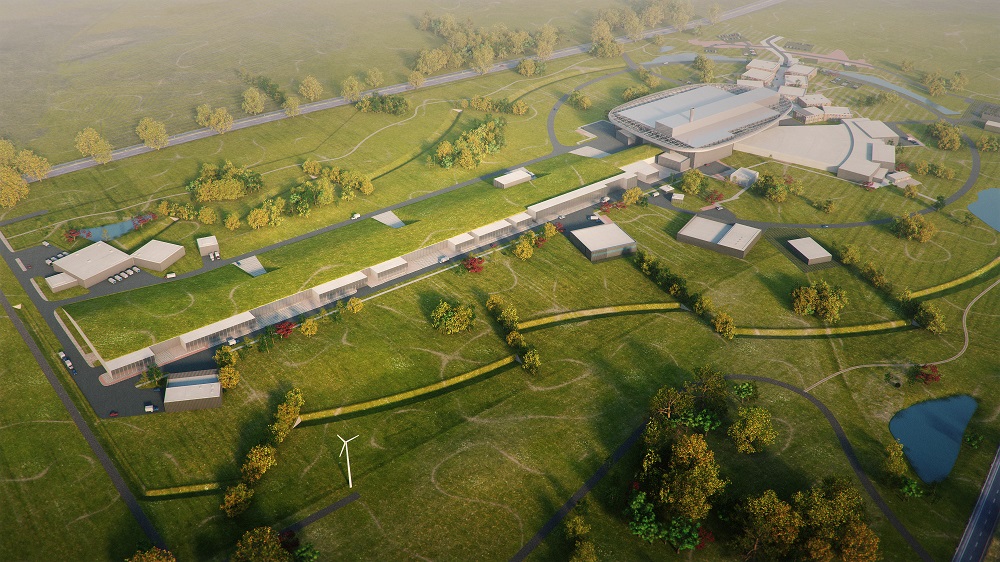
Spallation is a type of nuclear reaction in which the interacting nuclei disintegrate into a large number of protons, neutrons and other light particles, rather than exchanging nucleons between them. It is thought that most of the nuclei of light elements, such as boron, are made in this way. Spallation reactions of this type are thought to occur in interstellar space when a high-energy particle, such as a proton, hits a nucleus.
On Earth, at spallation sources, when a proton hits a nucleus, charged pions result. Pions can be positively or negatively charged or neutral. Pions (π) belong to the same class of subatomic particles of protons and neutrons, namely, hadrons. Hadrons are not truly elementary particles, as they consist of quarks: three in the case of protons and neutrons, or two in the case of pions, a quark and an antiquark. Charged pions decay into muons (μ) and, importantly, neutrinos (ν). Neutrinos exist in three forms, one in association with the electron, one with the muon and one with the tau particle. Each form has its own antiparticle.
At spallation sources, both π+ and π– are produced in proton-nucleus collisions in the target. While the resulting π– are efficiently absorbed by nuclei before they can decay, the produced π+ lose energy as they propagate in the target and will eventually decay at rest into π+→ μ+νμ, followed in close spatial vicinity by the decay of the positive muon into a positron, an electronic neutrino and a muonic antineutrino. Thus, three neutrino flavours are engendered for each π+ created.
Besides the obvious gain in statistics with respect to other neutrino sources, the use of neutrinos from pion decay at rest presents a clear advantage: the energy dependence of the flux is well-known in this case, and there is only room for systematic uncertainties a ffecting its normalization. This contrasts with the large uncertainties associated to conventional neutrino beams, where neutrinos are produced in the decay of pions and kaons in flight, and the determination of the neutrino spectral shape relies on Monte Carlo simulations.
Low-energy neutrinos can scatter off the atomic nucleus as a whole, via the weak neutral current. During this process the initial and final states of the nuclear target are indistinguishable, permitting a coherent contribution from all nucleons. The net result is a drastic enhancement to the cross-section for this type of neutrino interaction, roughly proportional to the square of the number of neutrons present in the target nucleus. The single observable from this so-called coherent elastic neutrino-nucleus scattering (CEνNS) is a recoiling nucleus, which generates a signal in a energy range diffcult to reach with most contemporary radiation detectors. Importantly, the three neutrino flavours engendered for each π+ created in a spallation source have essentially identical CEνNS cross section.
The upcoming European Spallation Source (ESS) sited in Lund, Sweden, provides an unprecedented opportunity to perform high-statistics CEνNS measurements. The ESS will combine the world’s most powerful superconducting proton linear accelerator with an advanced hydrogen moderator, generating the most intense neutron beams for multi-disciplinary science to date. It will also provide an order of magnitude increase in neutrino flux with respect to previous sources (or to planned ones), like the Spallation Neutron Source at Oak Ridge National Laboratory. This will facilitate CEνNS measurements not limited in their sensitivity to new physics by poor signal statistics, while still employing nonintrusive, compact neutrino detectors, able to operate without interference with ESS neutron activities.
Now a team of researchers has explored 1 innovative detector technologies maximally able to profit from the order-of-magnitude increase in neutrino flux provided by the ESS, along with their sensitivity to a rich particle physics phenomenology accessible through high-statistics, precision CEνNS measurements. The researchers have considered a suite of innovative detector technologies that originate in the fields of dark matter detection and double-beta decay searches.
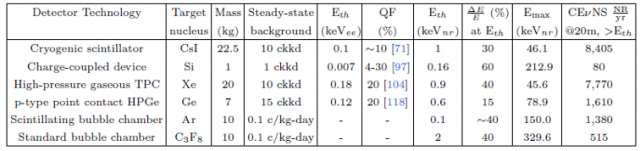
The researchers find that any of the detectors considered can lead to an improved precision in the determination of variables associated to a few representative new physics scenarios: non-standard neutrino interactions, weak mixing angle, neutrino charge radii and neutrino magnetic moments.
There is still time to incorporate any or some of these detectors before the scheduled start of ESS in 2023. Employing a variety of detectors can provide an enhanced sensitivity to neutrino properties, and the ability to confirm or refute any deviations from the Standard Model that might be observed via CeνNS.
Author: César Tomé López is a science writer and the editor of Mapping Ignorance
Disclaimer: Parts of this article may be copied verbatim or almost verbatim from the referenced research paper.
References
- D. Baxter, J. I. Collar, P. Coloma, C. E. Dahl, I. Esteban, P. Ferrario, J. J. Gomez-Cadenas, M.C. Gonzalez-Garcia, A. R. L. Kavner, C. M. Lewis, F. Monrabal, J. Munõz Vidal, P. Privitera, K. Ramanathan & J. Renner (2020) Coherent elastic neutrino-nucleus scattering at the European Spallation Source J. High Energ. Phys. doi: 10.1007/JHEP02(2020)123 ↩