Non-trivial biological quantum effects
Quantum mechanics and biology are very different fields. The first one studies things that are very small, like atoms, molecules or particles. Biology, on the other hand, deals with complex and big systems, the living beings. Of course, that does not mean that there is no connection at all. Big, complex systems are composed always by small simple components. It is not a secret that all animals and plants are formed by atoms and molecules. Also, chemical reactions can be described using quantum mechanics, and no one doubts about the importance of chemistry in biology.
A different thing is finding non-trivial quantum effects in biological systems. Quantum mechanics predicts many non-intuitive behaviors that we do not observe in our everyday life. One of them is quantum superposition, the fact that a quantum system can be in two different states at the same time. A second one, quantum entanglement, implies that in composite systems one part of them affects the other one immediately even if both parts are separated by millions of kilometres. This two phenomena have been observed in quantum systems, like cold atoms or photons, but we never see them in our macroscopical world. Why not? If we are composed by atoms, why can we not see superpositions of ourselves?
There are different possible answers to this problem, one is called the quantum-to-classical transition, but the most accepted one is that the interaction with our environment steals our “quantumness”. Even for small systems, like atoms, detecting these effects requires very specific equipment in order to isolate them from the environment. Usually, very low temperatures (below -200 ºC) and pressure (close to perfect vacuum) are necessary for the experiments, and this is not the usual environment of living beings. Because of that, quantum mechanics and biology have remained quite independent fields until recently. Just in the last years new experimental data hint to the possibility of real quantum superposition and entanglement during some biological processes. Reviewing all this possible effects is the goal of a paper recently published in Nature Physics 1.
Quantum coherent energy transport in photosynthesis
Photosynthesis is a multi level and very complex process. It is also very important, it maintains almost all life on Earth. Also, it is for sure the most popular topic in the field of quantum biology since, in 2007, Engel et al presented an spectroscopical analysis of the Fenna-Matthews-Olson (FMO) complex of green sulfur bacteria 2 . This complex has an important role in the photosynthetic process. Basically, it mediates the transfer of the energy that is captured by the photoreceptors, also called the antenna, to the reaction center, where the energy is transformed into useful chemical products. This transport happens with almost 100% efficiency, meaning that the energy of every single photon that is absorbed by the antenna ends in the reaction center. This is an important fact, as the green sulphur bacteria lives very deep in oceans and could be receiving one photon per hour, or even less, so a high efficiency is vital for their survival opportunities. In Figure 1, a small description of the green sulfur bacteria photosynthetic system is displayed.

The experiment from Engel et al showed that, at very low temperatures and by the use of laser pulses, the excitation travels across this complex in a very interesting way. Instead of just jumping from molecule to molecule until it is absorbed by the reaction center, one can see how the probability of finding the excitation in one of the molecules oscillates with time. These oscillations are a typical behavior of quantum systems, and suggest that there should be quantum coherence inside the systems. If that is true, it means that the excitations travels by different paths at the same time in order to find its destination. Of course, real bacteria do not live at very low temperatures, nor are they illuminated by lasers. The first problem was solved just recently by repeating the experiments at room temperature 3, the second one remains open.
Two things are unusual in this process. First, it has a very high efficiency, even being surrounded by a wet and hot environment. Second, it shows oscillations that can be related with quantum superposition. One strong researching line nowadays is to probe if there is any relation between these two things, and wether it is quantum superposition what makes those systems that efficient.
Avian compass and spin chemistry
Another interesting topic of study is avian magnetoreception. It is common knowledge that birds can detect magnetic fields and that they use this ability in their migrations. Before the invention of GPS birds had the most precise navigation system on Earth. The origin of such impressive ability is still not completely understood. There are several different theories about it, and one of them includes quantum effects. This is the radical-pair mechanism. This theory explains many of the behavioral studies performed on some species of migrating birds, and it implies a chemical reaction that should take place in the retina of birds, allowing them to “see” North.
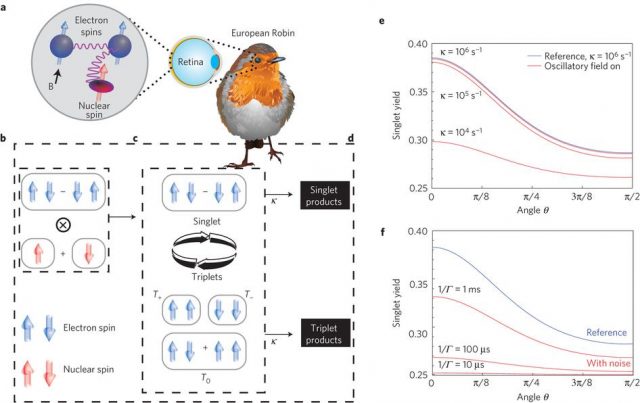
This kind of reaction is based on the spin of the electrons and nuclei. This “spin” is a quantum property, similar to a small compass that can point only to a certain number of points, in opposition to classical compass that can point in an arbitrary direction. Of course, as it is a magnetic property, spin is affected by an external magnetic field such as the one from Earth. The spins of electrons can determine if a certain reaction takes place of not, and as they are affected by magnetic fields ,the output products of this reaction can be used as a compass.
So, what are the quantum effects here? Well, the spin is a pure quantum property, with no classical counterpart, but there is more. Spins can be found in different configurations, but always abiding by a rule called the Fermi Exclusion Principle, that makes some of the allowed configurations entangled, meaning that separated electrons are correlated in their spins. Also, it has been proved that this entanglement can survive or even be revived by the interaction with the environment 45. In Figure 2 , there is a description of this model for the European robin, together with a plot of the concentration of the chemical products depending on the traveling angle of the bird with respect to North.
For this effect there are not a direct experiment involving real birds, but there are many other experiments that show the dependency of certain chemical reactions on the external magnetic fields. More specific experiments should follow in order to determine the correct mechanism of the avian compass.
Photosynthesis and avian magnetoreception are without any doubt the most popular topics in quantum biology, but there are other phenomena candidates to be based in quantum mechanics. Examples are the tunneling effect in the olfactory system and photoreceptors in the visual one. Reference [1] includes a more technical and complete review.
Quantum effects in biological systems is a novel and interesting field of study. Biological systems are very efficient and complex, and there are evidences that indicate that they may also be quantum. It is also common knowledge that quantum systems can perform tasks that are impossible for classical ones, mainly in communication and computing. Maybe, these two facts are related and, through evolution, Nature has created devices that are extremely efficient as they exploit quantum behaviours. Only time, and experiments, can clarify this point.
References
- Lambert, N. et al Quantum Biology. Nature Physics 9, 10–18 (2013) DOI: 10.1038/nphys2474 ↩
- Engel, G. S. et al. Evidence for wavelike energy transfer through quantum coherence in photosynthetic systems. Nature 446, 782–786 (2007) ↩
- Panitchayangkoon, G. et al. Long-lived quantum coherence in photosynthetic complexes at physiological temperature. Proc. Natl Acad. Sci. USA 107, 12766–12770 (2010) ↩
- Gauger, E. M. et al. Sustained quantum coherence and entanglement in the avian compass. Phys. Rev. Lett. 106, 040503 (2011) ↩
- Cai, J. et al. H. J. Quantum control and entanglement in a chemical compass. Phys. Rev. Lett. 104, 220502 (2010) ↩
2 comments
Pretty interesting post!
It reminded me that an article about FRET http://en.wikipedia.org/wiki/Förster_resonance_energy_transfer and its uses in biology could be really interesting for those who are not familiar with such concept.
Foster energy transfer is very popular in photosynthesis research. It was the accepted by the community process in light harvesting. The point is that recently a more complicate process, with quantum coherence, has been detected in this kind of systems, and that is what created this boom on quantum biology.