Irradiating food waste for energy production
Author: Daniel González-Muñoz is a predoctoral researcher in photocatalytic processes at Universidad Autónoma de Madrid.
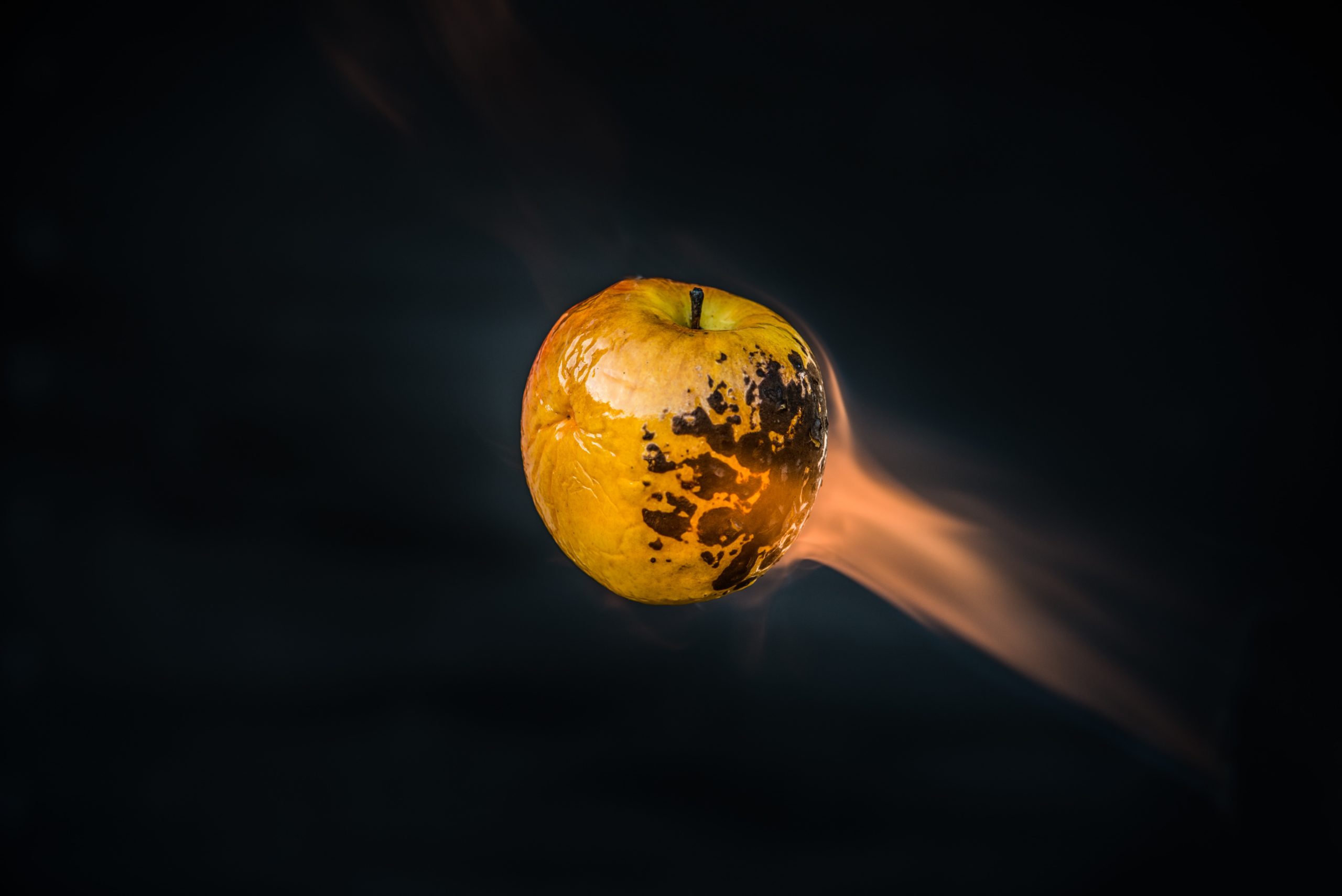
“Eat all your food, it can’t be wasted”. How many times have you heard that phrase from your parents? We did not know it, but they were right. Nowadays, a third of the food we generate is wasted. This generates both economic losses (~ $940 billion USD per year) and environmental problems (4.4 Gt of CO2 per year, 8% of total greenhouse gases). Many of these losses can be avoided by improving market infrastructure. However, even if the goal set by the United Nations to cut food losses in half by 2030 is met, billions of tons of food waste will still be misspent. Therefore, the development of new technologies that can address this problem has become a prominent topic in scientific research.
In this line, photoreforming (PR) is presented as a very promising alternative. This procedure combines the exploitation of food waste and the production of clean fuel. In PR, a semiconductor is excited by light (hν), promoting an electron from the valence band (VB) to the conduction band (CB) (Figure 1a). Then, two processes occur simultaneously: an electron (e–) from the CB reduces water to form H2 as fuel, while holes (h+), generated in the VB are able to carry out the oxidation of organic substrates (Figure 1b). This cooperative system has several benefits, such as applicability in a small off-grid system, compatibility with mixed and wet waste, ability to produce pure H2, and only sunlight energy is necessary. The PR principle has been reported with simple organic molecules, sugars or plastics, but their study in food waste is limited. The few works based on the oxidation of food waste use a TiO2 semiconductor, a very common photocatalyst that absorbs ultraviolet (UV) light. Photocatalysts with UV absorption limit the use of sunlight due to the low amount of it in the solar spectrum (5%). For this reason, photocatalysts that absorb across a wider range of the solar spectrum (visible and infrared light) need to be developed.
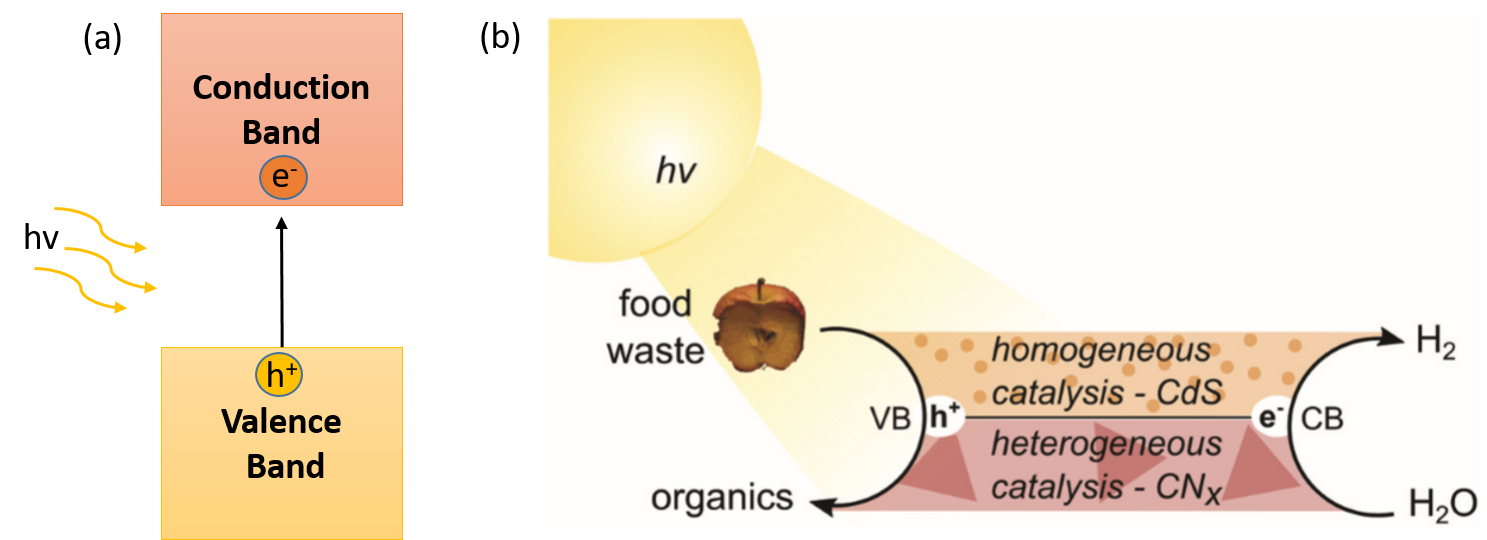
For this purpose, the authors developed two different photocatalysts 1. One is based on quantum dots of cadmium sulfide and cadmium oxides (CdS/CdOx), whose nanostructures are water-soluble nanocrystals less than 10 nm in size that absorb in the solar spectrum below 515 nm (Figure 2a). The other photocatalyst is formed by carbon nitride doped with Ni2P as co-catalyst (H2NCNx|Ni2P). This structure is a heterogeneous (non-soluble) photocatalyst that absorbs in the solar spectrum below 460 nm (Figure 2b-c).
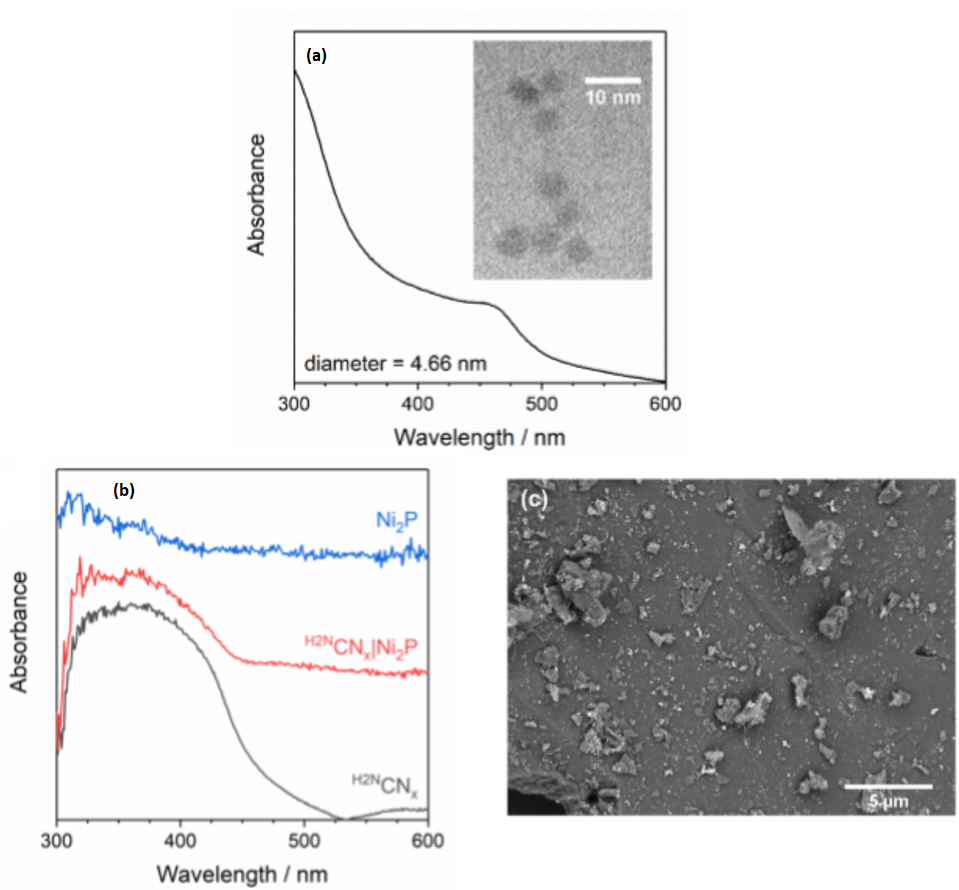
A preliminary study was carried out analyzing the production of H2, under simulated solar light irradiation, from fructose and starch at different pH levels. The results showed that CdS/CdOx is capable of generating more H2 than H2NCNx|Ni2P. However, H2NCNx|Ni2P is capable of producing H2 at alkaline, neutral and acidic pH, while CdS/CdOx can only do so under alkaline conditions. The next step was to analyse H2 production with more types of food waste, under simulated solar light irradiation for 20 h (Figure 3). Due to the versatility of H2NCNx|Ni2P in different pH ranges, and the development of the reaction conditions as environmentally friendly as possible, the experiments for this photocatalyst were carried out in neutral pH (Figure 3b). The simplest substrates, such as sugars or glycerol, are more soluble in water and gave better yields in H2 production. In addition, CdS/CdOx gave better yields in H2 production (Figure 3a) than H2NCNx|Ni2P (Figure 3b). This is because CdS/CdOx is a homogeneous, water-soluble photocatalyst, therefore charge transfer with the substrates is more effective, even when the substrates are insoluble. Furthermore, CdS/CdOx absorbs over a wider range of the solar spectrum (λ<515 nm vs λ<460 nm for H2NCNx|Ni2P), which helps it take better advantage of solar radiation. The system can be optimized since it was shown that after 5 days of irradiation the system continued to produce H2.
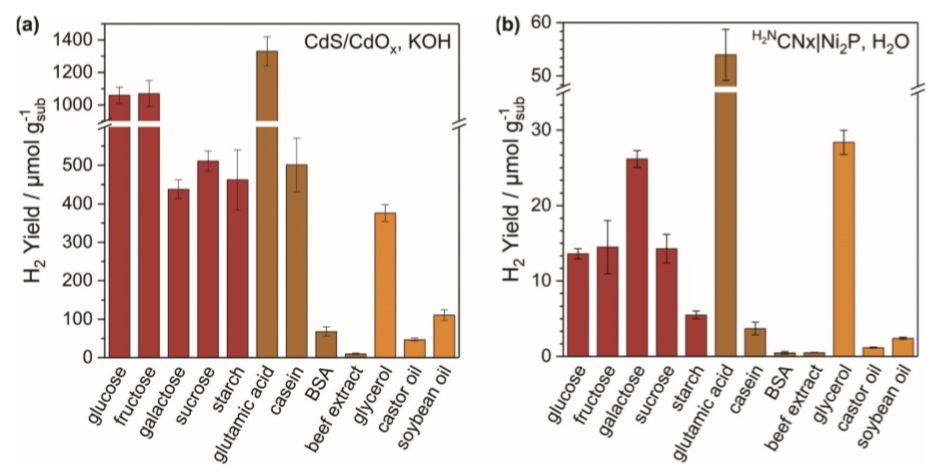
So far, we have seen how H2 is produced in this photocatalytic system, but what about substrates which undergo oxidation? A multitude of oxidation by-products were detected by nuclear magnetic resonance (NMR) and high-performance liquid chromatography (HPLC). As an example of an oxidation by-product, figure 4 shows the detection of formate in the oxidation of casein, fructose and starch. As you can see, the formation of formate is higher with CdS/CdOx because of the advantages that we mentioned above related to this photocatalyst.
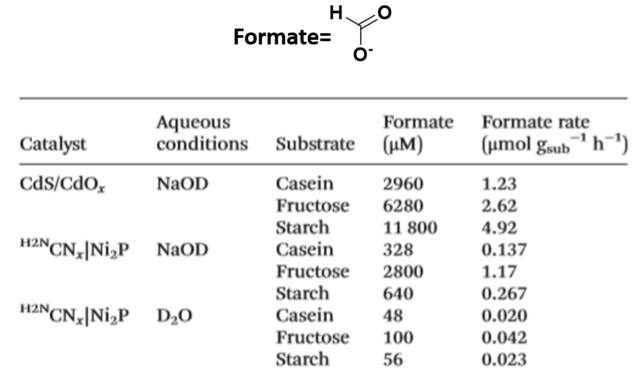
Finally, the PR concept was applied to real food waste. Three of the most discarded products in the UK were used: apples, bread and cheese. For all three products, CdS/CdOx is the photocatalyst that produces the best yield in H2 generation. On the other hand, H2NCNx|Ni2P is capable of generating more H2 under alkaline conditions, showing a moderate yield in H2 production under neutral conditions (Figure 5a). One of the biggest problems in food waste management is its incompatibility with mixed waste. In this regard, an attempt was made to take the PR one step further, using artificial mixtures and real-world municipal waste (Figure 5b). As expected, CdS/CdOx presented the best results in H2 production, while H2NCNx|Ni2P produced moderate yields in both alkaline and neutral media (Figure 5b, inset).
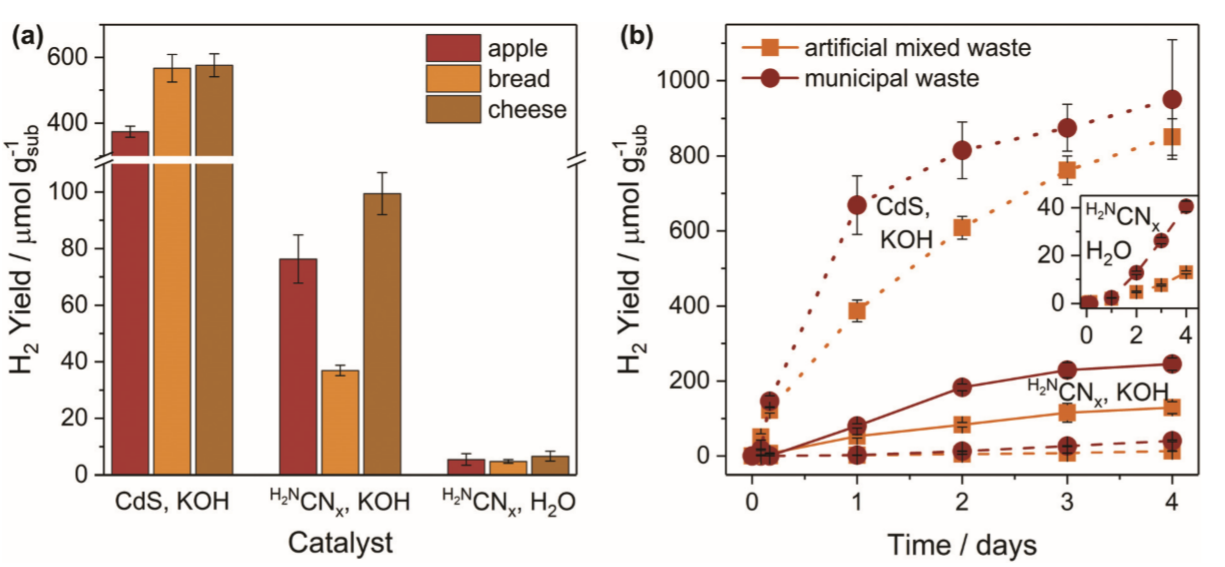
The results obtained for the PR process are very promising. However, it has a major drawback today. The CO2 footprint is very high (⁓55 000 g CO2 per kW h H2), due to the use of KOH in the medium, the electrical energy to stir the reaction and the energy used in the pre-treatment of food waste. However, we should not view these results as negative. In an ideal scenario – in which a neutral medium would be used – with a 100% conversion to H2 and formate while using renewable energy to generate electrical energy and to stir and heat the system, the carbon footprint would be -3200 g CO2 per kW h H2.
These results show that photoreforming is presented as an alternative with many options in the management of mixed food waste. Further study and improvement of reaction conditions, which could reduce the CO2 footprint, would make photoreforming a very efficient way of both producing clean energy and managing food waste at the same time.
References
- Taylor Uekert, Florian Dorchies, Christian M. Pichler, Erwin Reisner (2020) Photoreforming of food waste into value-added products over visible-light-absorbing catalyst. Green Chemistry. 22, 3262-3271. doi: 10.1039/d0gc01240h ↩