Temporal dedifferentiation to solve neurodegeneration
Author: José R. Pineda got his Ph.D. from University of Barcelona in 2006. Since 2007 he has worked for Institut Curie and The French Alternative Energies and Atomic Energy Commission. Currently he is a researcher of the UPV/EHU. He investigates the role of stem cells in physiologic and pathologic conditions.
Alzheimer’s disease (AD) is a condition that gradually damages a person’s memory and ability to think clearly, eventually making it difficult to handle even simple daily tasks. Symptoms of Alzheimer’s usually start showing up in older adults, typically those over 65. According to World Health Organization (WHO), AD is the most common cause of dementia, accounting for as many as 60-70% of senile dementia cases and affecting 47.5 million people worldwide 1. What’s important to know is that the changes in the brain that cause Alzheimer’s can start many years—even a decade or more—before any symptoms become noticeable. Early damage often occurs in areas of the brain essential for memory, such as the hippocampus and the entorhinal cortex. During this early stage, harmful changes take place in the brain, including the buildup of proteins that cluster into “plaques” and “tangles”. These clusters prevent brain cells from working properly. The discovery of the degeneration of cholinergic neurons and the reduction of acetylcholine levels in postmortem studies of patients resulted in the use of drugs that lead to the increase of acetylcholine levels in brain 2. As the disease progresses, more areas of the brain are affected, and the brain itself begins to shrink. By the final stages, damage is widespread, and the brain tissue has decreased significantly in size.
The development of new effective drugs involves a difficult and time-consuming process, accompanied by a very high failure rate. For this reason, alternative strategies are urged. It is estimated that number of people with neurodegenerative disorders will reach five million by 2050 [2]. The collaborative work done at the Vall d’Hebrón, University of Barcelona, Max-Plank and Inserm among others, had the brilliant idea of using Yamanaka factors (YFs), known to dedifferentiate and reverse some aging features in mammalian tissue, in the mouse brain in a controlled spatiotemporal manner to increase the number of neurons and glia, preventing the development of progressive cognitive decline 3. Some previous ideas paved the way 4, however, let us see how they arrived to get the astonishing results…
To put in context, the YFs are four transcription factors that have the ability to reprogram somatic cells (specialized cells of our body) to a pluripotent state (a type of stem cell able to give rise to new or other specialized cells). To date, YFs have been used to reverse age-associated phenotype of muscle, skin and other tissues 56, but very little data exists about their role in central nervous system, due to their prolonged expression in mouse brain lead to the formation of tumoral masses and animal death 7. However, the authors decided to do a controlled spatiotemporal induction of YFs in the mouse brain across two different scenarios: 1) during brain development and 2) in adult stages within the context of AD neurodegeneration.
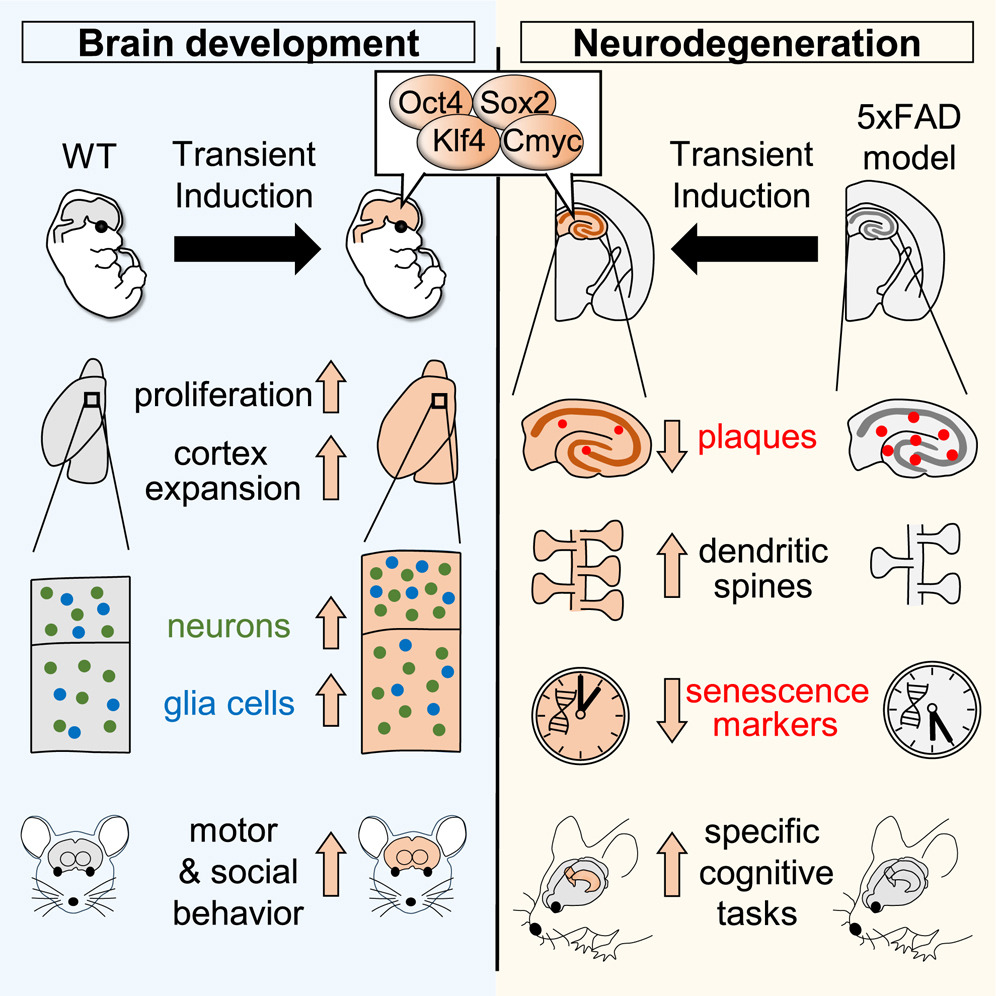
First of all, they decided to unravel what happened during transient YF induction. To do that they used a double genetically engineered mouse model for the system of expression: the Tet-ON system (that is the tetracycline transactivator (rtTA), a system based on the regulatory elements that control the activity of the tetracycline-resistance operon in bacteria) and the content to express (the inducible-four factors (i4F) model carrying a single copy of the i4F polycistronic cassette). In this way, the authors were able to allow the induction of YFs thanks’ to the administration of Doxyciclyne (Dox) in the drinking water. To find the effects during brain development, the authors administered Dox in the drinking water or pregnant females and after 4 days sacrificed and analyzed the brains of the embryos. They found that the treatment led to bigger brains and the elongation of the germinal layer (region with neurogenic niches). Furthermore they observed invaginations of the ventricular surface (take in mind that a normal mouse brain has a relatively smooth surface with no folds (it is known as “lissencephalic”). In contrast, the best example of a brain with many invaginations is the human brain (it is known as “gyrencephalic”)). Unfortunately, animals were not viable due to impaired liver hematopoiesis, so the authors decided to reduce Dox dose. Histological analyses revealed that YF induction was able to transiently perturb cell identity co-expressing Sox2 (progenitor marker) and Ctip2 (neural marker) and increase cell proliferation (PH3 staining and EdU pulse labeling are two complementary techniques to identify proliferating cells) during development.
After finding that YF induction perturbed cell identity and increased cell proliferation, they decided to do a “clean” mode to have a nervous-system-specific induction of YFs. For that, they used Nestin-Cre animal (Nestin is particularly expressed in brain during animal development). Using this approximation, they circumvented the liver hematopoiesis problem, allowing high Dox doses or longer treatments. They found much bigger brains, with an expanded cortex and a weight up to double that of control littermates. To find what was happening at intracellular level, they analysed the transcriptomic profiles and cell composition by single-cell RNA sequencing (scRNAseq) and they found the upregulation of the major clusters related to cortical development with more cells in the S and G2/M phases (characteristic of mitosis). Astonishingly, they found that the cortical expansion persisted into adulthood, with more neurons, astrocytes and oligodendrocytes and enlarged cortical layers II–IV of both prefrontal and motor regions in 5-month-old mice. Thus, next step was to check behavioral tests. They found that the YF mice showed an enhanced ability to maintain their balance, an improved motor performance and had less impulsive behavior than WT mice. Remarkably, using the three chamber social interaction test 8 YF mice showed an increased sociability index respect to control animals.
Finally, having observed that the transient YF induction is tolerated by post-mitotic neurons and the behavioral improvement in cognitive skills, the authors hypothesized that a similar protocol could be applied to a pathologic scenario of animals carrying a neurodegeneration. To do that, they did the YF induction driven by synapsin-1 (SYN1)-dependent rtTA-expressing adeno-associated virus (AAV) injected into the dorsal hippocampus of 8-week-old (in this way, the YF expression was restricted to the neurons, due to are the only cells that have synapsin-1). The host animals selected to receive the AAV were the 5xFAD mice model of AD. Treatments were done 3 days per week from 12 to 35 weeks. They evaluated structural plasticity of spine density in neuronal dendrites (the spines are very small regions were synapsis occurs) and presynaptic and postsynaptic changes using electron microscopy to determine a rescue of their levels in 5xFAD AVV treated mice. From these results, they concluded that intermittent YF induction not only was tolerated by adult hippocampal neurons but induced synaptic improvements in the 5xFAD mouse model. Furthermore, they quantified the amyloid plaque load and found a strong reduction with high interaction of the microglia with the plaques, suggesting that with better neurons the microenvironment is also improved but to a lesser extent due to no changes in astrocyte reactivity (reduction of astrogliosis) were observed. Despite the limited results, the authors prosecuted conducting behavioral tests evaluating emotional and cognitive skills. The plus mace (anxiety test) and forced swimming test (test to determine animal mood) gave no improvements but the evaluation of cognitive flexibility and spatial memory in a T-maze resulted in an improvement. More strikingly, the novel object location test demonstrated that treated animals rescued the preference, lost in non-treated 5xFAD animals. All these results concluded that despite YF induction in the dorsal hippocampus of the 5xFAD mice ameliorated some of the cognitive deficits, it was not enough to restore their emotional alterations.
In conclusion, the intermittent expression of YF was compatible with the preservation of neuronal identity, becoming a safe approach that led to cognitive, molecular, and histological improvements in the 5xFAD mice model of AD. Furthermore, transient YF induction as a powerful tool for modulating neural proliferation, and it may open new therapeutic strategies for brain disorders.
References
- Revi M. Alzheimer’s Disease Therapeutic Approaches. Adv Exp Med Biol. 2020;1195:105-116. doi: 10.1007/978-3-030-32633-3_15 ↩
- Dubois B, Feldman HH, Jacova C, Hampel H, Molinuevo JL, Blennow K, DeKosky ST, Gauthier S, Selkoe D, Bateman R, Cappa S, Crutch S, Engelborghs S, Frisoni GB, Fox NC, Galasko D, Habert MO, Jicha GA, Nordberg A, Pasquier F, Rabinovici G, Robert P, Rowe C, Salloway S, Sarazin M, Epelbaum S, de Souza LC, Vellas B, Visser PJ, Schneider L, Stern Y, Scheltens P, Cummings JL. Advancing research diagnostic criteria for Alzheimer’s disease: the IWG-2 criteria. Lancet Neurol. 2014 Jun;13(6):614-29. doi: 10.1016/S1474-4422(14)70090-0. ↩
- Shen YR, Zaballa S, Bech X, Sancho-Balsells A, Rodríguez-Navarro I, Cifuentes-Díaz C, Seyit-Bremer G, Chun SH, Straub T, Abante J, Merino-Valverde I, Richart L, Gupta V, Li HY, Ballasch I, Alcázar N, Alberch J, Canals JM, Abad M, Serrano M, Klein R, Giralt A, Del Toro D. Expansion of the neocortex and protection from neurodegeneration by in vivo transient reprogramming. Cell Stem Cell. 2024 Oct 17:S1934-5909(24)00327-8. doi: 10.1016/j.stem.2024.09.013. ↩
- Olova N, Simpson DJ, Marioni RE, Chandra T. Partial reprogramming induces a steady decline in epigenetic age before loss of somatic identity. Aging Cell. 2019 Feb;18(1):e12877. doi: 10.1111/acel.12877. ↩
- Yang, J.H., Hayano, M., Griffin, P.T., Amorim, J.A., Bonkowski, M.S., Apostolides, J.K., Salfati, E.L., Blanchette, M., Munding, E.M., Bhakta, M., et al. Loss of epigenetic information as a cause of mammalian aging. Cell. 2023, 186, 305–326.e27. https://doi.org/10.1016/J.CELL.2022.12.027. ↩
- Mahmoudi, S., and Brunet, A. Aging and reprogramming: a two way street. Curr. Opin. Cell Biol. 2012, 24, 744–756. https://doi.org/10.1016/J.CEB.2012.10.004. ↩
- Abad, M., Mosteiro, L., Pantoja, C., Cañamero, M., Rayon, T., Ors, I., Graña, O., Megías, D., Domínguez, O., Martínez, D., et al. Reprogramming in vivo produces teratomas and iPS cells with totipotency features. Nature 2013, 502, 340–345. https://doi.org/10.1038/nature12586. ↩
- Yang, M., Silverman, J.L., and Crawley, J.N. (2011). Automated Three-Chambered Social Approach Task for Mice. Curr. Protoc. Neurosci. Chapter 8, Unit.8.26. https://doi.org/10.1002/0471142301.NS0826S56. ↩