Adipocytes orchestrate T-cell acute lymphoblastic leukemia propagation
T-cell acute lymphoblastic leukemia (T-ALL) is a devastating disease of T-cell progenitors that mainly affects children and young adults. Numerous genomic alterations are known to induce survival, proliferation, and differentiation of T-ALL cells 1. The interactions between leukemic cells and their microenvironment are known factors that contribute to T-ALL pathogenesis. 23456 The bone marrow (BM) microenvironment consists of numerous cellular components such as osteoblasts, endothelial sinusoidal cells, mesenchymal stromal/stem cells (MSCs) and hematopoietic cells. In addition, BM also contains adipocytes, which are differentiated cells dedicated to store triglycerides 7. This extramedullary adipose tissue can be classified into white and brown adipose tissue and adipocyte-poor and adipocyte-rich niches which in the literature has been commonly described 8. The adipocyte-poor BM is a primary site for hematopoiesis, meanwhile the adipocyte-rich BM is known to inhibit it 9. Besides, the adipocyte-rich BM is a dynamic tissue that grow in response to several types of injuries such as irradiation, chemotherapy or starvation stimulus 10. Recently, a correlation of the interplay between the presence of adipocytes and the growth of solid cancer has been identified 1112, the relationships between adipocytes and hematological malignancies are less known. For this reason, in the present study X. Cahu and collaborators investigate how adipocyte-rich or -poor BM sites control T-ALL development 13.
To answer this question, the authors compared leukemic infiltration in different sites of the BM mice and showed a delayed T-ALL cell infiltration and expansion in the adipocyte-rich tail BM compared with thoracic segments (adipocyte-poor BM). Thus, using flow cytometry, they next characterized T-ALL cells from different BM niches (thoracic, femur tail niches) in terms of cell-surface markers. hT-ALL cells displayed a distinct cell-surface phenotype in thoracic and tail BM, whereas femur- and thorax-derived cells were similar. Leukemic cells from the tail BM had a decreased forward and side scatter respect the rest of the cells (forward scatter measures the cell size and side scatter measures internal cell complexity (i.e. granularity)). Moreover, using flow cytometry also allows labeling cell-surface markers such as cell receptors. They also found that leukemic cells from the tail BM expressed significantly lower levels of CD7, CD1a, CD3, TCRab, CD4, CD8, CD49d, CXCR4, and CD31 receptors but increased CD44 receptors compared with thoracic BM, whereas thorax- and femur-derived T-ALL had comparable cell-surface marker levels. These niche-specific T-ALL differences could be induced either by the distinct microenvironment in tail/thoracic BM or as a result from the selection of distinct clones in those niches. To find out the right response between these two hypotheses, they performed a Comparative Genomic hybridization analysis (“CGH”, a technique used to detect the alterations in the genomic DNA sequence). They took samples of T-ALL cells isolated from thoracic and tail BM, however they did not appreciate any change or correlation between the more frequently deleted and mutated genes. Thus, T-ALL cells from thoracic or tail BM were phenotypically different but genomically similar, questioning the impact of the BM sites on T-ALL characteristics.
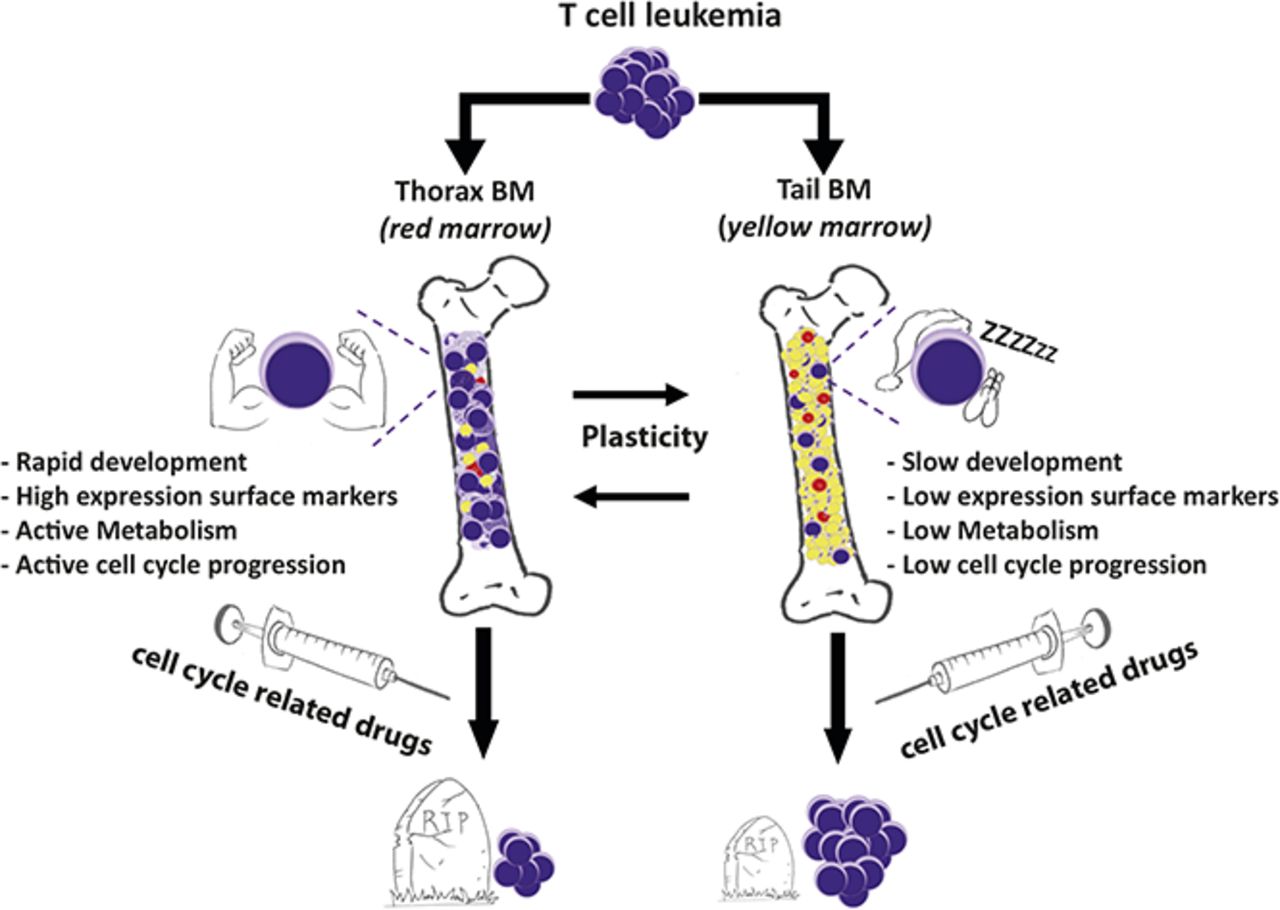
To track down the role of the microenvironment in T-ALL development, thoracic- and tail-derived T-ALL cells were isolated, and their growth was studied in identical ex vivo conditions. Short-term co-cultures produced lower cell growth of leukemic cells from tail-derived hT-ALL respect to thorax-derived hT-ALL cultures. This result suggested a short-term niche-specific imprints on T-ALL growth. The question of the niche imprints on T-ALL phenotype and growth was further explored after secondary transplants of xenografted cells isolated from the primary tail and thorax vertebrae. They observed that both thorax- and tail-derived T-ALL cells could infiltrate tail and thorax vertebrae and femurs. A kinetic of femur BM samplings indicated that tail-derived cells have a delayed infiltration ability compared with thorax-derived cells. Limiting dilution analysis showed a diminished ability of T-ALL to invade secondary BM femur. These characteristics resulted in prolonged survival of mice transplanted with tail-derived T-ALL.
Thorax- and tail-derived hT-ALL were next characterized in terms of gene expression using human-specific Affymetrix gene arrays (which allows whole genome gene expression analysis). Among the different activated pathways, the basal OXPHOS was significantly lower in tail compared with thorax-derived hT-ALL, suggesting tail-derived cells are resting. Interestingly, microarray analyses also pointed to glycolysis differences in T-ALL from thorax and tail BM. These results were confirmed by measuring the glycolytic capacity of the BM-derived T-ALL, with the glycolytic reserve being absent from tail-derived T-ALL. Gene array analyses also indicated an enrichment of E2F targets (which include genes encoding proteins involved in the initiation of replication, nucleotide metabolism, and the enzymatic synthesis of DNA) in thorax- vs tail-derived hT-ALL which is correlated with the fact that Thorax-derived cells were actively proliferating, whereas tail-derived cells were more frequently in the G0 state of the cell cycle. Finally, microarrays highlighted an increased activated apoptotic pathway in the tail-derived niche.
Because tail-derived hT-ALL cells display a decreased cell-cycle progression and a low metabolism, authors speculated that their intrinsic sensitivity to drugs might be different compared with thorax-derived hT-ALL cells. To probe this hypothesis, leukemic cells isolated from thoracic or tail vertebrae were maintained with different chemotherapeutic agents. They observed that tail-derived hT-ALL cells displayed a lower sensitivity to chemotherapeutic drugs compared with thorax-derived ones. When thorax- and tail-derived T-ALL cells were grown with a drug and transplanted at equal numbers in secondary immunodeficient mice, the animals that received drug-treated tail-derived cells succumbed 10 days before those injected with thorax-derived cells. This result put into evidence the lower tail-derived hT-ALL sensitivity to the drug.
Given that tail vertebrae is full of adipocytes, authors finally investigated whether another adipocyte niche would similarly imprint on T-ALL. They studied T-ALL infiltration in the gonadal adipose tissue (GAT) and observed that, as observed in tail BM, few leukemic cells were detected compared with any of the studied BM sites. Phenotype analysis outlined similar decreased surface marker expression in GAT- and tail-derived hT-ALL cells, that differ from femur- and thorax-derived hT-ALL. They also explored the adipocyte influence on leukemic cell behavior during T-ALL co-cultures in the presence of adipocytes derived from the differentiation of stromal MS5 cells. It is well-known that leukemic cells need to be maintained in co-culture with MS5 cells for optimal grow ex vivo. Interestingly, they observed that T-ALL cells poorly grow upon co-cultures with adipocytes. Because BM adipocytes might protect hT-ALL from chemotherapy, they assessed T-ALL sensitivity to a chemotherapeutic drug during co-cultures with MS5 or MS5-derived adipocytes. Using this approach, they observed that drug-mediated apoptosis was lower when leukemic cells were grown with MS5-derived adipocytes compared with MS5 cells. This result demonstrated that BM adipocytes may take part in hT-ALL chemoresistance observed in the adipocyte-rich tail BM.
In summary, authors demonstrated that BM sites differentially orchestrate T-ALL propagation stamping specific features to leukemic cells such as quiescence and decreased response to chemotherapy.
Author: Marta Irigoyen is a postdoctoral researcher at CIC bioGUNE
References
- Van Vlierberghe P, Ferrando A. The molecular basis of T cell acute lymphoblastic leukemia. J Clin Invest 2012; 122: 3398-3406. PMID: 23023710 DOI: 10.1172/JCI61269. ↩
- Pitt LA, Tikhonova AN, Hu H, Trimarchi T, King B, Gong Y, Sanchez-Martin M, Tsirigos A et al. CXCL12-Producing Vascular Endothelial Niches Control Acute T Cell Leukemia Maintenance. Cancer Cell 2015; 27: 755-768. PMID: 26058075 DOI: 10.1016/j.ccell.2015.05.002. ↩
- Uzan B, Poglio S, Gerby B, Wu CL, Gross J, Armstrong F, Calvo J, Cahu X et al. Interleukin-18 produced by bone marrow-derived stromal cells supports T-cell acute leukaemia progression. EMBO Mol Med 2014; 6: 821-834. PMID: 24778454 DOI: 10.1002/emmm.201303286. ↩
- Silva A, Laranjeira AB, Martins LR, Cardoso BA, Demengeot J, Yunes JA, Seddon B, Barata JT. IL-7 contributes to the progression of human T-cell acute lymphoblastic leukemias. Cancer Res 2011; 71: 4780-4789. PMID: 21593192 DOI: 10.1158/0008-5472.CAN-10-3606. ↩
- Armstrong F, Brunet de la Grange P, Gerby B, Rouyez MC, Calvo J, Fontenay M, Boissel N, Dombret H et al. NOTCH is a key regulator of human T-cell acute leukemia initiating cell activity. Blood 2009; 113: 1730-1740. PMID: 18984862 DOI: 10.1182/blood-2008-02-138172. ↩
- Winter SS, Sweatman JJ, Lawrence MB, Rhoades TH, Hart AL, Larson RS. Enhanced T-lineage acute lymphoblastic leukaemia cell survival on bone marrow stroma requires involvement of LFA-1 and ICAM-1. Br J Haematol 2001; 115: 862-871. PMID: 11843820 DOI: 10.1046/j.1365-2141.2001.03182.x. ↩
- Rosen ED, Spiegelman BM. What we talk about when we talk about fat. Cell 2014; 156: 20-44. PMID: 24439368 DOI: 10.1016/j.cell.2013.12.012. ↩
- Tavassoli M, Crosby WH. Bone marrow histogenesis: a comparison of fatty and red marrow. Science 1970; 169: 291-293. PMID: 4247471 DOI: 10.1126/science.169.3942.291. ↩
- Naveiras O, Nardi V, Wenzel PL, Hauschka PV, Fahey F, Daley GQ. Bone-marrow adipocytes as negative regulators of the haematopoietic microenvironment. Nature 2009; 460: 259-263. PMID: 19516257 DOI: 10.1038/nature08099. ↩
- Sheng X, Mittelman SD. The role of adipose tissue and obesity in causing treatment resistance of acute lymphoblastic leukemia. Front Pediatr 2014; 2: 53. PMID: 24926474 doi: 10.3389/fped.2014.00053. ↩
- Manabe Y, Toda S, Miyazaki K, Sugihara H. Mature adipocytes, but not preadipocytes, promote the growth of breast carcinoma cells in collagen gel matrix culture through cancer-stromal cell interactions. J Pathol. 2003; 201: 221-228. PMID: 14517839 DOI: 10.1002/path.1430. ↩
- Herroon MK, Rajagurubandara E, Hardaway AL, Powell K, Turchick A, Feldmann D, Podgorski I. Bone marrow adipocytes promote tumor growth in bone via FABPdependent mechanisms. Oncotarget. 2013 Nov;4(11):2108-2123. doi: 10.18632/oncotarget.1482. PMID: 24240026 ↩
- Cahu X, Calvo J, Poglio S, Prade N, Colsch B, Arcangeli ML, Leblanc T, Petit A et al. Bone marrow sites differently imprint dormancy and chemoresistance to T-cell acute lymphoblastic leukemia. Blood Adv 2017; 1: 1760-1772. PMID: 29296822 DOI: 10.1182/bloodadvances.2017004960. ↩