Zooming through graphene: The strange world of electron transit time
Imagine sending a tiny particle, like an electron, through a material so thin it’s just a single layer of atoms. You’d expect it to take a certain amount of time to pass through, right? Maybe a little longer if the material gets thicker, or shorter if it’s super thin. But what if the electron seemed to zip through faster than it should, or even appeared to arrive before it was supposed to? That’s the kind of mind-bending puzzle physicists are tackling in a fascinating study about electrons moving through graphene and similar materials. Let’s dive into a new study 1 which reveals a phenomenon called “negative transit time.”
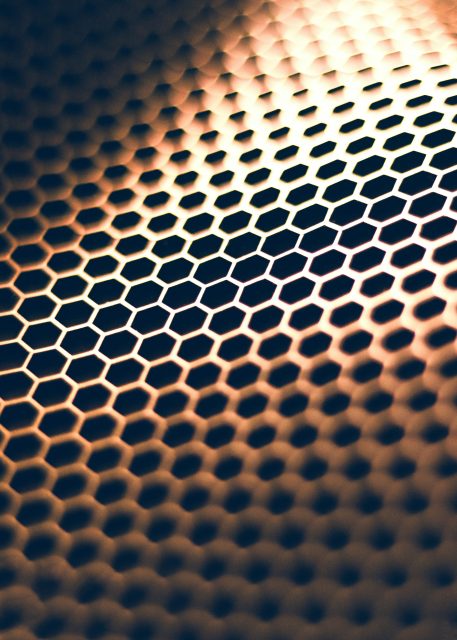
Graphene is a remarkable material made of carbon atoms arranged in a honeycomb-like pattern, forming a sheet just one atom thick. It’s incredibly strong, conducts electricity well, and has sparked excitement in fields from electronics to nanotechnology. In this study, researchers focused on how electrons behave when they pass through graphene and other ultra-thin materials, like hexagonal boron nitride (h-BN) and even a single layer of oxygen atoms. They weren’t just interested in whether the electrons made it through, but in how long it took them to do so. What they found challenges our everyday intuition about time and motion, showing that electrons can sometimes act as if they’re breaking the rules of physics.
To understand this, let’s start with the concept of transit time. When an electron moves through a material, we can think of its journey as having a start and an end, like a runner crossing a finish line. Scientists measure how long it takes for the electron to go from one side of the material to the other. This time isn’t just about the physical distance; it’s influenced by how the electron interacts with the atoms in the material. In the quantum world, where electrons behave like both particles and waves, these interactions can lead to some surprising outcomes.
Measruring the Wigner Time Delay
One way to measure this transit time is through something called the Wigner time delay. Don’t worry about the name—it’s just a way to compare how long an electron takes to pass through a material versus how long it would take if it were moving freely through empty space. If the electron gets held up by the material, the delay is positive. If it somehow seems to speed up, the delay can be negative. This study found that in certain cases, the Wigner time delay in graphene and similar materials becomes not just negative, but dramatically so, suggesting the electron is moving faster than expected—or even arriving before it should.
How does this happen? The key lies in the way electrons scatter, or bounce around, as they move through these thin materials. Imagine throwing a ball through a room filled with mirrors. The ball might hit a mirror and change direction, taking a longer path to the other side. Electrons in a material like graphene can scatter in similar ways, but in this case, the scattering happens in the plane of the material—what the researchers call “in-plane scattering.” This is different from scattering that happens as the electron moves straight through from one side to the other. The in-plane scattering creates extra paths for the electron, like side roads that branch off the main highway.
The researchers used a mix of advanced calculations and a simpler model. They used a method called augmented-plane-wave scattering theory to simulate how electrons move through graphene with high accuracy, taking into account the material’s atomic structure. They also created a basic two-dimensional model to show that the negative transit time is a general feature of thin materials, not just a quirk of graphene. This model helped explain why the effect happens: the interference of electron waves, caused by in-plane scattering, creates a kind of “fast-forward” effect at certain energies.
The researchers discovered something astonishing: at specific energies, when new scattering paths (called secondary diffraction beams) open up, the electron’s transit time can become negative. This doesn’t mean the electron is literally traveling backward in time, but rather that the wave-like nature of the electron leads to interference effects that make it appear to exit the material sooner than it entered. It’s as if the electron’s wave pattern is reshaped in a way that pushes its “arrival” forward in time. This effect is particularly strong in single-layer materials like graphene, h-BN, and oxygen, where the simplicity of the structure amplifies these quantum quirks.
A new twist on the Hartman effect
To make sense of this, the researchers compared the electron’s behaviour in different scenarios. In thicker stacks of graphene, the transit time behaves more predictably, sometimes increasing with thickness or staying constant in certain energy ranges, much like what happens in traditional materials. But in a single layer, the in-plane scattering creates a dramatic effect. At a critical energy point, where these new scattering paths emerge, the transit time doesn’t just dip slightly—it plummets, becoming hugely negative. This is a new twist on an older idea called the Hartman effect, where particles tunnelling through a barrier seem to take a fixed amount of time regardless of the barrier’s thickness. Here, though, the electrons aren’t tunnelling; they’re passing through in a way that’s allowed by classical physics, yet still producing bizarre results.
A path to better experiments
Why does this matter? For one, it could help scientists design better experiments to study electrons in materials. The researchers suggest using a technique called laser streaking, where a laser pulse tracks the electron’s motion with incredible precision, down to attoseconds (that’s a billionth of a billionth of a second). By studying how electrons move through graphene, scientists can learn more about the material’s properties and how to use it in technologies like super-fast electronics or quantum computers. The negative transit time also offers a way to manipulate electron waves without losing too much of their intensity, which could be useful for controlling signals in nanoscale devices.
Bigger questions
This discovery also connects to bigger questions in physics. For decades, scientists have puzzled over how to define the time it takes for a quantum particle to move from one place to another. The negative transit time in graphene suggests that our classical ideas about time and motion don’t always apply in the quantum world. It’s a reminder that at the smallest scales, nature plays by rules that can seem downright strange.
For anyone fascinated by the frontiers of physics, this study is a glimpse into the weird and wonderful behaviour of electrons in cutting-edge materials. It shows that even in something as simple as a single layer of atoms, there’s a universe of surprises waiting to be explored. The negative transit time isn’t just a curiosity—it’s a clue to understanding how quantum particles move and interact, and it might just help us build the technologies of tomorrow. So, the next time you hear about graphene, think of those electrons zipping through, defying time in ways we’re only beginning to understand.
Author: César Tomé López is a science writer and the editor of Mapping Ignorance
Disclaimer: Parts of this article may have been copied verbatim or almost verbatim from the referenced research paper/s.
References
- E. E. Krasovskii and R. O. Kuzian (2025) Negative Transit Time in Nontunneling Electron Transmission through Graphene Multilayers Phys. Rev. Lett. doi: 10.1103/PhysRevLett.134.126302 ↩