The Sandia Z machine unveils the interior of gas-giant planets
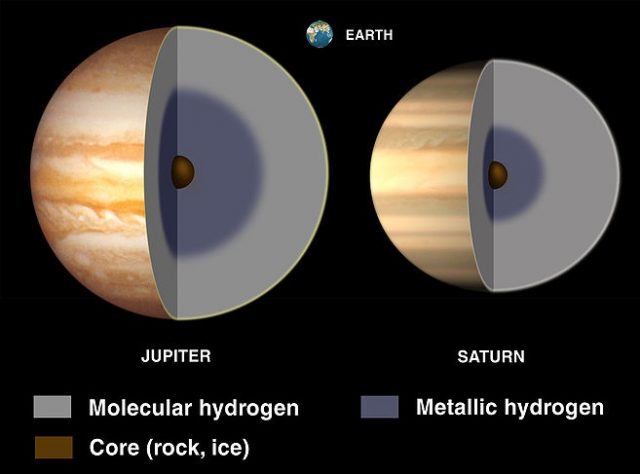
The standard three-layer model for the interior of Jupiter and Saturn claims that an outer layer of molecular hydrogen surrounds an inner layer of liquid metallic hydrogen, with a probable rocky, molten core. The Z machine at Sandia National Laboratories has been used to explore the boundary between the insulator and metallic hydrogen layers. At very large pressure, the molecular hydrogen shows a liquid-liquid, insulator-to-metal transition (LL-IMT) into metallic hydrogen. Markus D. Knudson, Sandia National Laboratories, Albuquerque, New Mexico, USA, and colleagues have published in Science the first direct observation of this transition in dense liquid deuterium at pressures around 300 gigapascals (GPa) and temperatures between 1000 and 2000 K during a tenth of a microsecond 1. Under these conditions, the Z machine reproduces in the laboratory the high pressures due to the gravitational field inside the gas-giant planets.
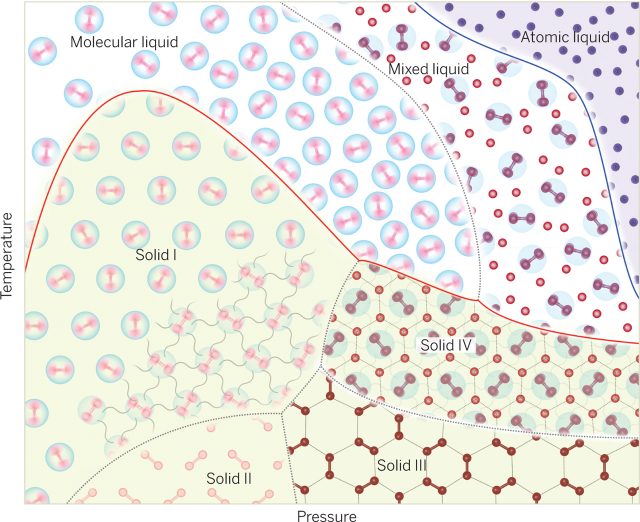
The most famous conjecture in condensed-matter physics still awaits a proof 2. In 1935, Hillard Huntington and Eugene Wigner calculated the properties of hydrogen squeezed to high density and pressure 3. They predicted that under pressures above 25 GPa, hydrogen would undergo a density-driven transition from an insulating, molecular solid to a conducting, atomic solid. Current experiments up to pressures of 250 GPa have not yet observed such transition. Figure 2 shows a schema of the pressure-temperature (PT) diagram of the four well-established solid phases using a schematic. Phase I is a hexagonal close-packed molecular liquid, where H2 molecules are rotating behaving as if they have a spherical shape. As pressure increases and temperature decreases a broken symmetry phase II appears, where the rotation stopped. But if temperature increases, around 900 K and 70 GPa, the solid melts into a molecular liquid phase.
Under further pressure increase, according to theoretical simulations and suggested by experiments, groups of three hydrogen molecules arrange themselves into hexagonal trimers. Figure 2 illustrates that at low-temperature results in phase III, where all molecules are in such trimers, but at high temperature appears phase IV, showing alternating layers of trimers and relatively freely rotating molecules. The four phases of solid hydrogen are nonmetallic. However, Huntington and Wigner conjecture, the appearance of a metallic solid phase of hydrogen has yet been produced neither in the theoretical simulations nor in the experiments.
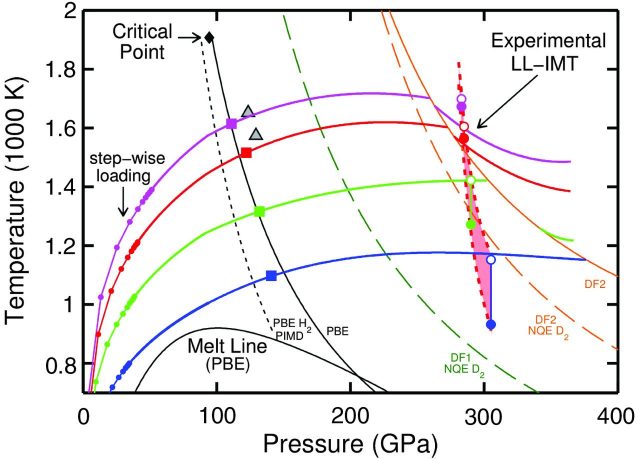
The liquid phases of hydrogen shown in Figure 2 have been diagnosed by Knudson et al. [1] by using deuterium, since at high temperatures the difference between deuterium and hydrogen is very small. The Z machine uses an aluminum plate propelled by giant capacitors to generate electrical shock waves in a tiny sample of deuterium. Essentially they look for the reflection of visible light at the interface between the deuterium sample and its aluminum holder. Figure 3 summarizes the results. There is a first strong signal drop in reflectivity around 120 GPa, associated to a nonmetallic state. But at a pressure between 280 to 305 GPa, the reflected light reappears indicating that the liquid hydrogen has turned into a shiny metal. Theoretical simulations confirm this interpretation of the result. This result shows that metallization occurs at lower pressures in the liquid than in the solid, at least at high temperature.
Figure 3 compares the theoretical LL-IMT phase boundaries and the experimental PT paths followed in the work by Knudson et al. [1]. The shaded red region indicates the experimentally determined location of the LL-IMT. Sandia Z machine generates intense electrical pulses (~20 million amperes) with large magnetic field densities (~10 million gauss) reaching magnetic pressures of several hundred GPa within a low inductance load. To diagnose the liquid deuterium samples the reflectivity of a 532-nm laser light is measured using a spectrometer (450- to 650-nm bandwidth). The experiments show a dramatic increase in reflectivity of the deuterium samples which is interpreted as an evidence of an abrupt density-driven LL-IMT. This interpretation of the experimental results require its comparison with those of computer simulations obtained by means of density functional theory (DFT). The reasonable agreement is not enough to definitively state that this transition is first-order, an abrupt transition, but the detailed analysis of the observations done by Knudson et al. [1] suggests that the transition is indeed first-order.
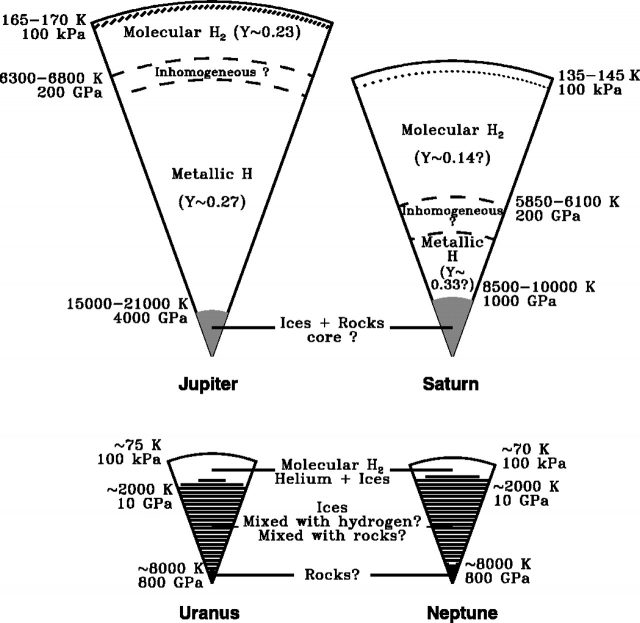
From the point of view of planetary science, the results of Knudson et al. [1] are relevant because the measured pressure for the LL-IMT in hydrogen at low temperature is larger than predicted by current computer simulations. They considerably underestimate it. Consequently, estimates for the low-pressure boundary of the region of hydrogen-helium immiscibility, which have been calculated using these computer simulations, are likely also considerably underestimated. The new measurement of the metallization pressure of hydrogen in the warm dense liquid suggests that the low-pressure boundary of the immiscibility region should be at or above ~300 GPa. This boundary location will affect the fractions of Saturn and Jupiter that are thought to lie within the immiscibility region and will likely alter our understanding of the structure and evolution of these and other gas-giant planets.
In fact, the new measurement points to a solution of a widely known puzzle in our current understanding of Saturn, it is significantly brighter than predicted for its age 4. The giant planets of our Solar System radiate more energy than they receive from the Sun. Current theoretical models predict well the infrared luminosity of Jupiter, but show the Saturn anomaly. First-principles calculations suggest that hydrogen metallization acts as a catalyst for hydrogen-helium demixing due to its immiscibility. This phenomenon results in an additional energy source though latent heat associated to rains of helium-rich droplets. Existing calculations of Saturn’s evolution based on a pressure for LL-IMT in hydrogen about 200 GPa suggest that this energy released by helium rains might be insufficient. However, the new measurement of the pressure by Knudson et al. [1] yields a higher value, cf. about 300 GPa, which may solve the Saturn’s puzzle and play an important role in our understanding of the evolution of the gas-giant planets.
References
- M. D. Knudson, M. P. Desjarlais, A. Becker, et al., “Direct observation of an abrupt insulator-to-metal transition in dense liquid deuterium,” Science 348: 1455-1460, 26 June 2015, doi: 10.1126/science.aaa7471. ↩
- E. Wigner, H. B. Huntington, On the possibility of a metallic modification of hydrogen. Journal of Chemical Physics 3: 764-770, 1935, doi: 10.1063/1.1749590. ↩
- Graeme J. Ackland, “Bearing down on hydrogen,” Science 348: 1429-1430, 26 June 2015, doi: 10.1126/science.aac6626. ↩
- Jérémy Leconte, Gilles Chabrier, “Layered convection as the origin of Saturn’s luminosity anomaly,” Nature Geoscience 6: 347-350, 2013, doi: 10.1038/ngeo1791. ↩
1 comment
[…] More @ mappingignorance.org […]