An almost luminical spin for a supermassive black hole
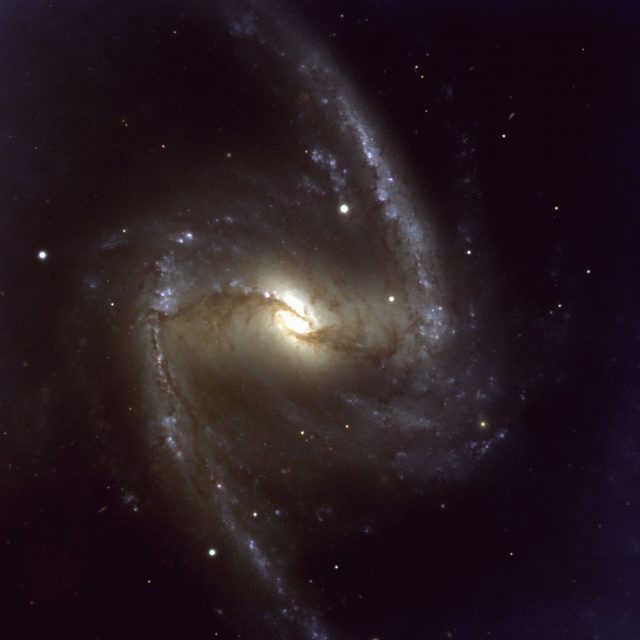
NGC1365 is a beatiful galaxy. It is big and bright enough to be observed with a medium-sized amateur telescope. Sometimes mis-located at the southern constellation of Eridanus, it is indeed located in the smaller Fornax constellation and it is one of the most notable peers in a famous galaxy cluster. Being one third of the full Moon in size, its main counterback is that it is usually located too low in the sky when observed from northern mid-latitudes. But, if you are lucky enough to travel to lower latitudes and enjoy a dark sky, you will find an amazing spectacle when observing the also called Great Barred Galaxy.
However, this galaxy is not simply a nice view through an eyepiece. It is also a clue to understanding how the black holes behave. Bright and relatively close galaxies (we are ‘only’ 56 million light-years from NGC1365) give us the chance to observe in detail some aspects that would remain elusive otherwise. And rotation of the central black hole is arguably one of the most intriguing ones.
When you observe the inner region of an active galaxy at X-ray wavelengths, you find broad emission lines from neutral and partially ionized iron. Those emission lines have been usually assumed to be broadened by the extremely fast rotation of the inner accretion disk. The distorted shape of such emissions can be caused by relativistic effects in the neighbourhood of a rotating black hole. This implies that rotation really matters when you are close enough to a black hole. By detecting the X-ray emissions, we are sounding the innermost possible region in such a dangerous place, the so-called ‘innermost stable orbit’. And how far this is from the black hole itself depends strongly on the spin rate of the supermassive object. The Big Bang Theory fans and theoretical-physics-heads should probably refer to the classic paper by Kerr 1.
Unfortunately, and as usual, devil is in the details. In fact, you normally cannot distinguish between fast rotation and absorption by other structures in the accretion disk. If you want to do so, you need more information than it is usually available. And here is where the paper recently published by Risaliti and collaborators comes to scene 2.
They used observations by the European Space Agency’s X-Ray Multi-Mirror Mission (XMM-Newton) and the NASA’s Nuclear Spectroscopic Nuclear Array (NuSTAR) telescope, both space-based missions. Those data provided the required temporal and spectral resolution to record the spectral variability of the inner accretion disk in the required few-hour timescales. By using data from both spacecrafts, they were able to extend their spectral coverage from 3 keV up to 79 keV.
I think that anybody involved in numerical modeling of natural events would appreciate the discussion presented by Risaliti. This is all about science: depending on how much information you have from a physical system you will have a number of opposing hypothesis clashing for explaining observations. However, as data grow they start showing deviations between models and, eventually, you will be lucky enough to discriminate. In their Nature paper, they show how models diverge as energy from iron emissions increases and so NuSTAR observations become the key to understand which of the two models is more likely (see Figure 2). The multiple absorber model has to be ruled out and relativistic reflection becomes the most plausible explanation for what we see.
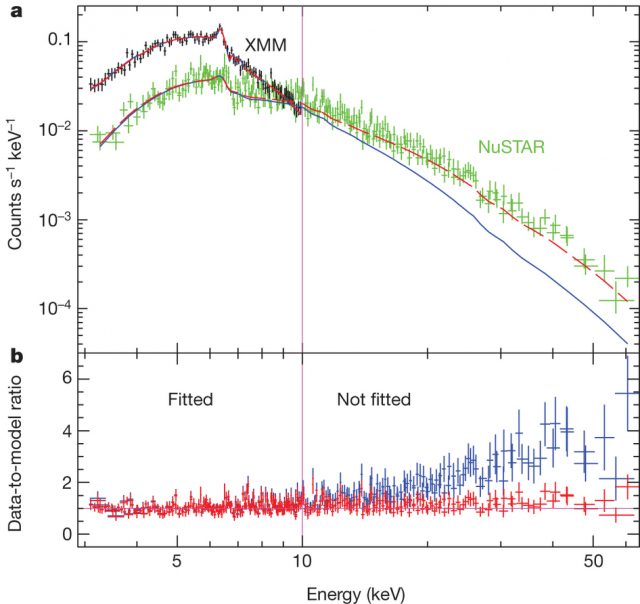
Great. So how fast is the spin of the black hole? We could say that its surface is rotating almost luminically, about 84% of light speed. This is possibly the first time anybody is able to measure the fast rotation of a super-massive black hole 3. That sounds impressive, since mass and spin is everything you need to know about a black hole. But wait and read, there is more.
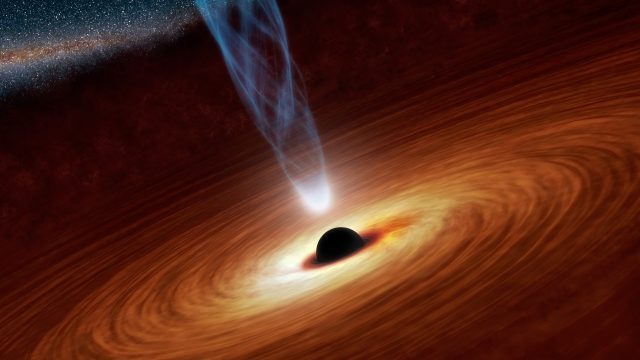
How can anything in the Universe get so fast? It is commonly accepted that galactic black holes were once younger and slimmer. As they ate the material accumulated in the accretion disk they grew bigger and bigger. But whether or not this means faster and faster depends on how they were fed by the surrounding medium. If the material is accreted irregularly, then the angular momentum is not expected to be so huge. An ‘ordered accretion’, instead, at the approppriate rythm will provide enough spin to the black hole. This is at least what Risaliti proposes in the companion press release [3].
Finally, a story on almost-luminical black holes has ended as a history of galaxy evolution. The authors of the paper say they can go even farther, but we still have to wait until data are analyzed. So let’s turn again to our telescopes to enjoy the view of sparkling galaxies that have so much to tell us.
References
- R. Kerr 1963. Gravitational Field of a Spinning Mass as an Example of Algebraically Special Metrics. Phys. Rev. Lett. 11, 237–238. d.o.i.: 10.1103/PhysRevLett.11.237 ↩
- G. Risaliti, F.A. Harrison, K.K. Madsen, D.J. Walton, S.E. Boggs, F.E. Christensen, W.W. Craig, B.W. Grefenstette, C.J. Hailey, E. Nardini, D. Stern and W.W. Zhang 2013. A rapidly spinning supermassive black hole at the centre of NGC1365. Nature, vol. 494, pp. 449 – 451. d.o.i.: 10.1038/nature11938 ↩
- Supermassive black hole spins super-fast. Harvard-Smithsonian Center for Astrophysics Press Release. Release number 2013-07. http://www.cfa.harvard.edu/news/2013/pr201307.html ↩