How spores get their boarding passes
Many organisms, such as fungi and plants without seeds, produce spores as a dispersal mechanism. Spores are usually microscopic propagules, many of them are able to resist harsh environmental conditions and successfully germinate after a long period of time. We know that their size is so small (just a bunch of micrometers) that they can effectively be transported by winds and global atmospheric currents up to gigantic distances, potentially even crossing oceans and continents. One may think that spores are a very efficient dispersal mode, and indeed they are, but we tend to forget that a change in the scale often implies a change in how physical mechanisms affect objects. In the particular case of spores, we find a paradoxical situation: while their tiny size allows them to be transported without limits by the wind, reaching the wind current itself constitutes an unexpected odyssey.
A spore-producing organism growing on the soil is actually “protected” from the winds by a layer of air subject to laminar flow. There is air movement within this layer, but if you really want to success dispersing your spores, your target is about 10 cm above, into the layer of turbulent air currents which, if you are lucky, may bring your spores far, far away. However, throwing microscopic objects even through humble distances is unexpectedly costly from our macroscopic perspective: air itself behaves as a sticky and viscous fluid if the projectile is too small, decelerating it very soon (to speak properly we should say that the Reynolds Number of a spore through air is very low). That is the paradox: you produce tiny propagules that can potentially “fly” to the other side of the planet, but you cannot make them “board” because of a ridiculous boundary of 10 cm of gooey quiet air. How do immobile organisms cope with this paradox? Producing thousands or millions of spores is a strategy to maximize the probability of any of them reaching a turbulent current by chance, but any mechanism allowing a spore-producing plant or fungus to eject their spores into the turbulence layer would be even better.
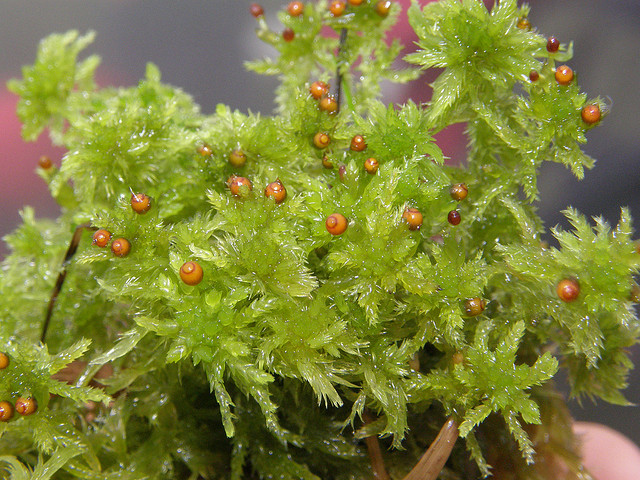
One of the most impressive mechanisms to overcome this circumstance can be found in peat mosses (genus Sphagnum), a diversified group of bryophytes with almost 300 species and a great ecological significance. Peat mosses produce tiny, spherical capsules of about 2 mm in diameter that are barely elevated over the level of the soil. During dry and warm days, when the capsules are mature, a patient observer can see how these capsules contract. The lid of the capsule eventually pops, unleashing a tiny yet powerful stream of spores that may reach up to 16 cm in height! This minute explosion is too fast for the human eye, but can be seen in detail with high-speed video.
Video 1. Ejection of spores by a single Sphagnum capsule, recorded at 250 fps and displayed at 15 fps | Credit: Nora Mitchell and Joan Edwards, uploaded by saperevedere
Video 2. High speed video of a Sphagnum capsule explosion, recorded at 10,000 fps and reproduced at 15 fps | Credit: Whitaker & Edwards (2010), uploaded by takowl
This phenomenon was already reported decades ago, but only recently started to be understood 1. The contraction of the Sphagnum capsule generates the incredible pressure of 200-500 kPa inside the urn. When the lid finally pops, the spores are projected at the very impressive initial speed of 16 m/s (more than 57 km/h). However, simple ballistics is not enough to explain the height reached by the spore stream: even a spore with such an initial speed would be completely stopped by air in less than half a millisecond after moving forward only about 7 mm. How do they reach that incredible distance? High-speed videos showed that the spore mass form a vortex ring, similar to the one observed in mushroom cloud of atomic explosions. Vortex rings are very well known in fluid dynamics, and one of their abilities is to self-propagate across long distances carrying the spinning fluid itself and, in this case, also the spores.
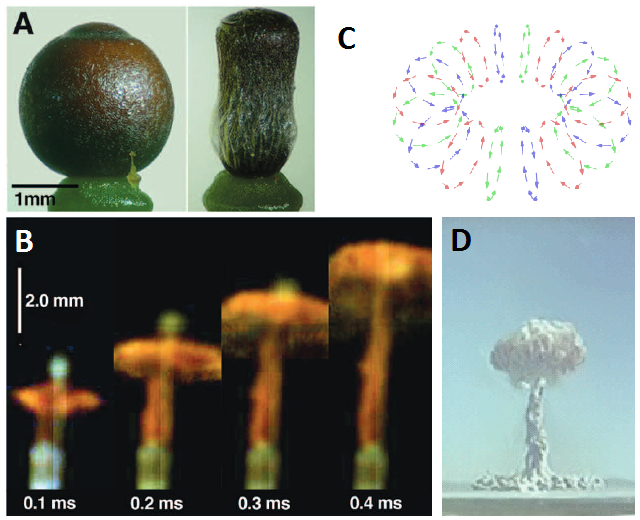
The vortex ring strategy is also known from some fungi that produce immersed fruiting bodies and also benefit from an effective ejection of their spores2. Other fungi produce a taller fruiting body, since elevating the spore-producing tissue even just some centimeters dramatically improves their dispersal success. Mushrooms are a good example: the stipe (“stem”) brings up the pileus (“cap”) so that the spores, produced underneath, just fall from the gills, and hence gravity is the mechanism that takes the spores out of the fruiting body. However, as we saw before, spores themselves are immobile, and since air currents cannot reach the spaces where they are produced (the gills of the pileus), and additional mechanism is required to perform this tiny leap. Most mushroom species use the “ballistospore” strategy3. Spores are produced by a cell called basidium, and they remain attached to it by a fragile filament called sterigma.
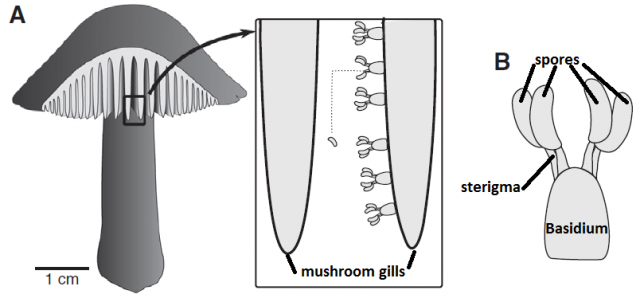
When the spore is ready to be fired, a microscopic drop of water will be generated by condensation near the junction of the sterigma and the spore. The drop is small enough to remain spherical thanks to its superficial tension, but it continues growing as more water molecules condense. When the drop diameter reaches a critical size, its superficial tension does not allow it to remain spherical anymore and it coalesces with the spore, triggering a violent shift of the center of mass and, ultimately, the ejection of the spore at 1.2 m/s, enough to jump out of the gill surface and let the gravity do the rest.
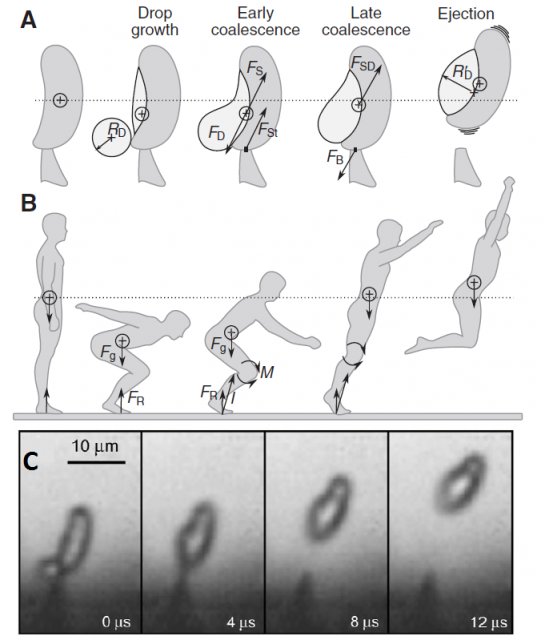
Video 3. Ejection of a ballistospore of the fungus Tilletia caries recorded at 24 fps. Now you see it, now you don’t. | Uploaded by wired
Finally, horsetails (a group of fern allies belonging to the genus Equisetum) also show a very creative strategy for spore movement. Unlike fungi or mosses, horsetails usually grow over the laminar flow layer, but they still need their spores to come out of the sporangium and be somehow thrown into potential air currents. Horsetail spores are very distinct: each of them has four appendages called elaters. The elaters are hygroscopic, meaning that they change their shape and position depending on the environmental humidity. The reaction of these structures to different conditions and a detailed reconstruction of their mechanism were recently addressed in a study4, concluding that there are two different functional consequences of elater function: Equisetum spores can walk and jump.
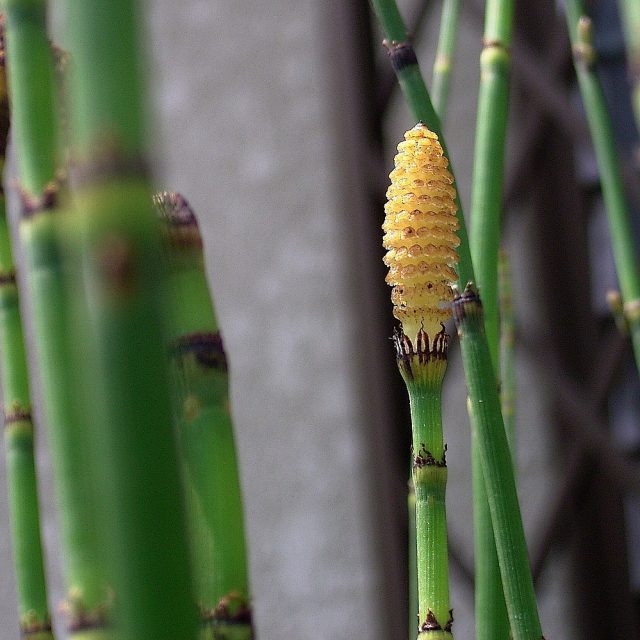
When the spore is subject to consecutive dry and humid cycles, the elaters expand and contract periodically, moving the spore a short distance in a random direction. In nature, these movements may be useful to get the spore out of the sporangium, reaching a free spot on its surface and exposing the spore to the atmosphere. These are the “walks”.
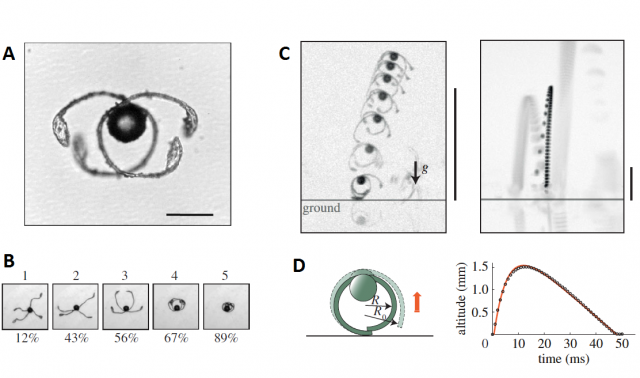
Sometimes, when the environmental humidity drops suddenly, two of the elaters get stuck, tangled, and they cannot unfold because of the friction between them. As the humidity continues dropping, more tension is accumulated in these elaters and eventually, they act like a spring and expand explosively, projecting the spore at about 1 m/s, allowing it to travel up to 1 cm, enough to leave the sporangium behind and “board” the flight of long distance dispersal.
Video 4. Walks and jumps of Equisetum spores recorded and explained by Philippe Marmottant. (Credit: Philippe Marmottant. Uploaded by UC2ctiTPRhrNZDwd0C1hdzrg
The bottom line of all these strategies is that the spores can efficiently overcome the small but yet overwhelming (for a microscopic particle) barrier that separates a sporangium or fruiting body from the air turbulences without any additional energy input, just taking advantage of the physic properties of fluids and surfaces at their own scale. Some of the authors of these works have remarked the potential of the study of these adaptations in the field of biomimetics in order to develop self-propelled objects or efficient microfluidic mechanisms.
References
- Whitaker, D. L. & Edwards, J. 2010. Sphagnum moss disperses spores with vortex rings. Science 329, 406–406 ↩
- Fritz, J. A., Seminara, A., Roper, M., Pringle, A. & Brenner, M. P. 2013. A natural O-ring optimizes the dispersal of fungal spores. Journal of The Royal Society Interface 10 ↩
- Noblin, X., Yang, S. & Dumais, J. 2009. Surface tension propulsion of fungal spores. Journal of Experimental Biology 212, 2835–2843 ↩
- Marmottant P., Ponomarenko A. & Bienaime D. (2013). The walk and jump of Equisetum spores, Proceedings of the Royal Society B: Biological Sciences, 280 (1770) 20131465-20131465. DOI: 10.1098/rspb.2013.1465 ↩