Flexible needles as the future for cancer treatment
With most of the conferences scheduled between June and October, beginning of the year brings with few novelties in robotics. During this time, roboticists must wait and wonder what the advances and “hot research topics” will be in their respective fields. Most likely, this year too one of those “hot” areas will be robotic insertion of flexible needles for minimally invasive surgery (MIS).
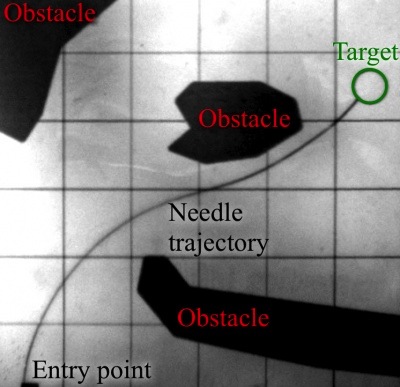
Compared to open surgery, MIS has better cosmetic outcomes and reduces trauma to patients, what results in shorter post operatory hospitalization and complications, with the obvious benefit to patient’s quality of life and cost savings. Cancer patients benefit from MIS too 1, in this case from the hand of percutaneous procedures. As can be guessed from its name, percutaneous procedures are those that access internal organs or structures through the skin, commonly with needles. In this approach, the doctor introduces a needle into a cancerous area to take a sample in case of a biopsy, or kills the cancer with the use of heat (heat ablation), cold (cryoablation) or a chemical substance (chemotherapy).
This is a difficult procedure for physicians, who can only place the needle with an error of 3 to 5 mm from the targeted point 2. It might seem a small error, but consider that the cancer is 10 to 20 mm in diameter. Error in needle placement means that a bigger area of tissue has to be killed to ensure that the whole cancerous area is destroyed. But can be worse in case of biopsies. If a sample is taken from healthy tissue instead of the suspected tissue, it can result in a false negative.
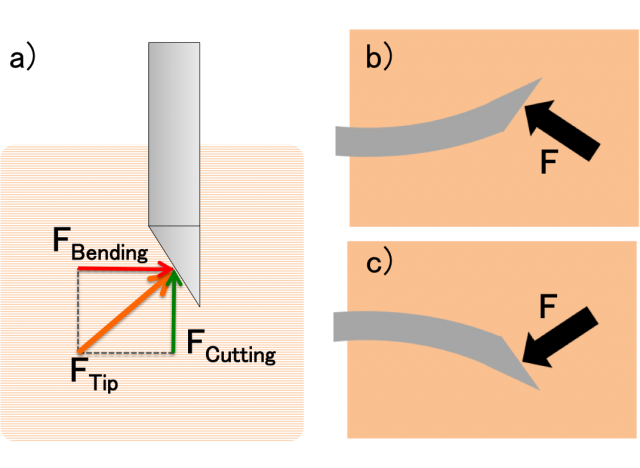
Tissue deformation caused by the needle and poor quality of ultrasound image are among the factors that contribute to this error, but more important for this discussion is needle bending. When a needle with a bevel tip is inserted into soft tissue, it will bend due to the asymmetry of the force applied on the tip (Figure 2). Initially, researchers tried to compensate for this effect by predicting the deformation of the needle, but in 2006, Webster et al. 3 proposed to use this effect to steer the needle in a desired direction. The idea is simple, if the needle deforms towards the opposite direction of the angled face, controlling its orientation one can control the direction in which the needle steers. This allows for more complex paths that can compensate for errors in needle trajectory, target displacement or avoid obstacles (Figure 1).
It must be noted that, although they are commonly referred to as needles, the truth is that they are made of nitinol wire (for biocompatibility) with a diameter of less than 1 mm. But right now clinical application is less important than to develop systems that can take advantage of the flexibility of needles to increase insertion accuracy. In the meantime, researchers cross their fingers hoping that in the future these “needles” can be of clinical use.
The model proposed by Webster et al. was based on the unicycle model for mobile robots (Figure 3). This is a common model used for bi-wheeled robots, like the Seagway, which can only move forwards and backwards or rotate over their vertical axis. Similarly, a needle can only be inserted or retracted and rotate around its central axis, equivalent to direction changes in a bi-wheeled robot. But this model has several limitations: it is constrained to two-dimensional trajectories, has constant bending radius and the predicted trajectory can be very inaccurate. Since then, other models have appeared that allow for 3D path planning, image-based trajectory control 4 or consider the needle as a beam with a load on the tip 5.
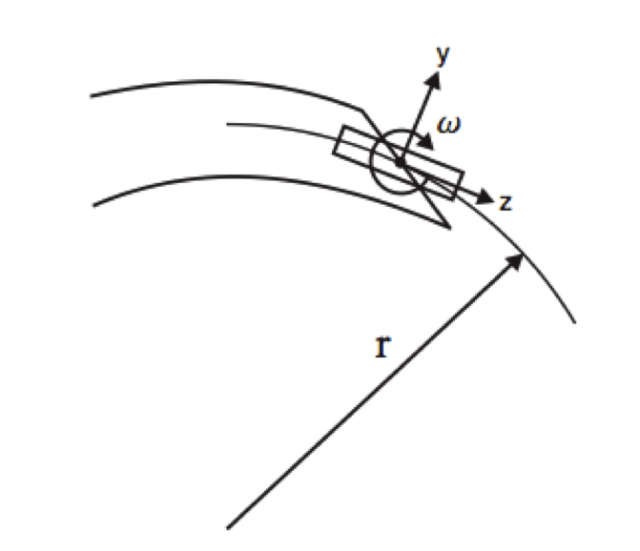
All these models have been validated at best using artificial phantom tissue. It is not uncommon to use phantom tissue in medical robotics to validate proposals, but these artificial tissues cannot replicate the complex behavior of living tissues. Generally they are made of silicone or gelatin that presents little variability in mechanical properties and no inhomogeneity, one big problem when experimenting with ex-vivo tissue, that not only changes properties from animal to animal, but also during experiments as it dries due to lack of blood irrigation.
If lack of clinical application wasn’t a concern, no proof of the steering capability of flexible needles in real tissue was a big one. Recently, Rucker et al. 6 published a variant of the unicycle model valid for 3D planning and more importantly, validated in phantom and ex-vivo porcine liver.
Compared to phantoms, flexible needles have a much bigger turning radius in real tissue. This means that they cannot bend as much and limits the complexity of the trajectories. To understand it better, imagine that you could turn the driving wheel of your car only 30 degrees to the left or right. It is clear then that the car wouldn’t be able to take sharp bends. The same effect happens to the needles in soft tissue. However, even if the needle can bend less the placement error is under 1 mm, what is an amazing and promising result, since the increase of accuracy provided by flexible needles might be more important than obstacle avoidance, which can be solved with careful trajectory planning.
There are still many open problems in robotic needle insertion, but there have been very promising results in recent years that let us think, that it might be possible to have automatic or semi-automatic needle insertion robots in the operating room in a not so distant future.
References
- S. Shiina, “Japanese experience in ablation therapies for hepatocellular carcinoma,” Hepatol Res., Sep 2007, 37 Suppl 2:S223-9 ↩
- E. M. Boctor, M. A. Choti, E. C. Burdette, and R. J. Webster, III, “Three- dimensional ultrasound-guided robotic needle placement: An experimen- tal evaluation,” Int. J. Med. Robot. Comput. Assist. Surg., vol. 4, no. 2, pp. 180–191, 2008 ↩
- R. J. Webster, III, J. S. Kim, N. J. Cowan, G. S. Chirikjian, and A. M. Okamura, “Nonholonomic modeling of needle steering,” Int. J. Robot. Res., vol. 25, no. 5/6, pp. 509–526, May/Jun. 2006 ↩
- R. Alterovitz, M. Branicky, and K. Goldberg, “Motion planning under uncertainty for image-guided medical needle steering,” Int. J. Robot. Res., vol. 27, pp. 1361–1374, 2008 ↩
- S.Misra,K.Reed,B.Schafer,K.Ramesh,andA.Okamura, “Mechanics of flexible needles robotically steered through soft tissue,” Int. J. Robot. Res., vol. 29, pp. 1640–1660, 2010 ↩
- Rucker D.C., Das J., Gilbert H.B., Swaney P.J., Miga M.I., Sarkar N. & Webster R.J. (2013). Sliding Mode Control of Steerable Needles, IEEE Transactions on Robotics, 29 (5) 1289-1299. DOI: 10.1109/TRO.2013.2271098 ↩
3 comments
[…] ¿Qué tiene que ver el Segway, ese sistema de transporte urbano con dos ruedas, con el tratamiento del cáncer? Aparentemente nada y sin embargo puede tener mucho en el futuro. Y es que precisamente se están desarrollando sistemas robóticos […]
[…] Zein da Segway, bi gurpilez hornitutako giza hiri-garraiagailua, eta minbiziaren kontrako tratamenduaren arteko harremana? Itxuraz bat bera ere ez eta etorkizunean asko izan dezakete. Izan ere, zauri txikiko kirurgian erabiltzeko, orratzak txertatzeko sistema robotikoak garatzen ari dira. Orain arte […]
[…] Flexible needles as the future for cancer treatment […]