The gravitational wave detector revisited: a new approach using atomic clocks
In September 2015 a new scientific field was born. Two colossal experiment confirmed the existence of gravitational waves, measuring the ripple of space-time induced by two very distant black holes merging in a cataclysmic display of gravity waves and radiation 1. Gravitational wave astronomy was born then, much like neutrino astronomy was born with the first detected neutrino back in 1956 (a work that earned those scientists a nobel price in 1995). A brand new way to look at the cosmos, enabling us to understand processes linked to the nature of reality itself. Since then a great number of different approaches to measure these waves are being proposed, catalyzing what was once a theoretical riddle into a thriving worldwide endeavor. Here I’ll talk about one of the last ideas: the use of atomic clocks to measure space-time waves.
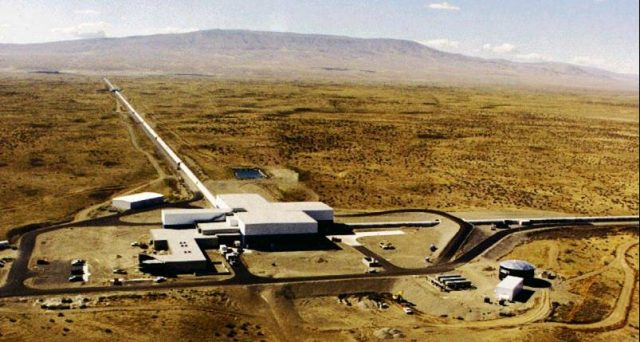
Gravitational waves were first proposed in the very late 19th century, but it was not until Einstein’s general relativity theory in 1916 that the idea gained momentum. They consist on ripples in space-time that can be generated by certain sources, propagating them outwards. If space-time is pictured as the surface of a lake, a gravitational wave would be the small height change that propagates outwards from an impact on the lake. But, since we’re dealing with space-time, the wave can change the distances between distant points, and also the time measured by two previously synced clocks. Put simply, if a gravitational wave traverses you right now, there can be a very subtle change in the distance between you and the screen that you’re looking at. This is produced by really heavy and compact objects moving around, like stars and black holes. Since the gravity force travels at a finite speed (the speed of light indeed), a change on the position of the source will propagate the change of gravity not instantaneously , but at the speed of light, much like a change of air pressure propagates at the speed of sound (and by generating complicated patterns of changing pressures with our throats we can talk).
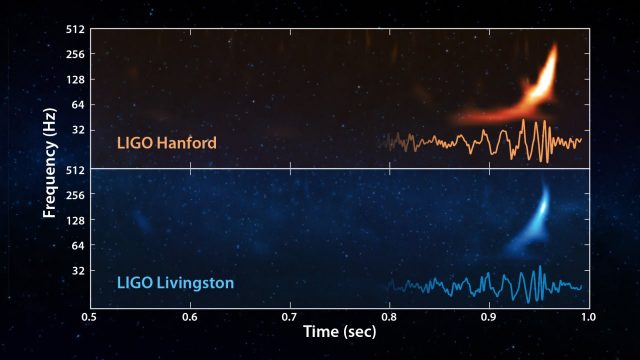
So let’s see how this would work. Picture that we have two spacecrafts somewhere in the space sharing an ultrastable laser between them. Each of these spacecrafts have it’s own optical lattice clock, an astonishingly precise atomic clock design. This design involves a number of atoms imprisoned in a lattice of some neutral material. A laser is fired upon them, exciting them and the light emitted when they go back to their natural state is measured as a frequency (ideally constant from the laws of nature), then transformed to tics in a clock. The catch is that this re-emitted light is fed back up on the laser to lock the frequency of it to the atom’s transition. This is, indeed, how the frequency is measured, by tracking how the feedback loop changes the laser frequency. And now imagine that we make both spacecrafts share their laser. Ideally both clocks should produce the same readout, but, what would happen if a gravitational wave changes the distance between both spacecrafts while a photon of that laser is traveling from one spacecraft to the other? Thing would be out of sync and the clocks would then produce different readouts, and we could understand this as a signal if we want to detect gravitational waves. And yes, those things are so incredible precise that with our current technology we would definitely see the influence of a gravitational wave in the setup.
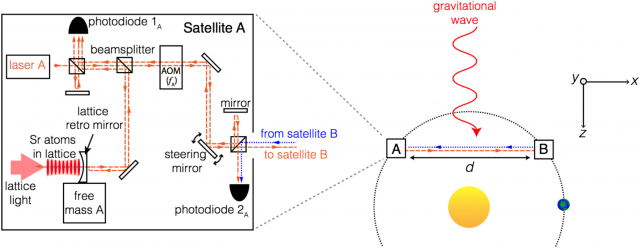
This is what was proposed by S. Kolkowitz and his peers in a recent paper from the university of Colorado and Cambridge, published in Physical Review Letters D 2. The optical lattice clock was just recently proven to be our most precise time measurement device and all sorts of applications are now being proposed all around the world. In the paper they do the numbers about the precision achievable with the design. In particular they propose a couple of drag-free satellites in heliocentric orbit (aka, around the Sun and not the Earth) separated by some big distance and connected by a single optical link. Each of those satellites harbors its own optical lattice clock (they consider a next-generation strontium-87 clock), and the results are quite interesting. This design does not outperforms the existing ones (LIGO or the still envisioned LISA), rather complements them. The “classic” design of gravitational wave detectors (to me sounds silly to call classic a thing like that) usually uses some sort of interference between lasers that traveled a great distance, sensing differences in the travel distance through differences in the interference pattern. This makes the design capable of sensing a broad band of gravitational waves frequency, but our new approach lack this feature because by its design it is locked to a particular frequency. So by design this experiment could be locked to a frequency where the future LISA detector would not be very sensitive, and by doing so keep track of a gravitational wave just where LISA cannot do anything. The team, then, proposes some kind of hybrid detector, convinced that merging both devices into the same system will not reduce the sensitivity of each one. They also talk about the technological need of understanding how optical lattice clocks can be space-grade manufactured (in particular the ultrastable laser part) if we want to put LISA in the space, making the atomic clock gravitational wave detector a reasonable pathfinder for that technology development.
In the end it seems that there is a fair interest on developing this idea. Its main drawback would be that it may need LISA to succeed, an already tight budget mission with never ending delays. Without the technological pathfinder point, and giving the already good sensitivities of current detectors compared to this proposal, it may fall short to financially interest research agencies.
References
- LIGO Scientific Collaboration and Virgo Collaboration; Abbott, B. P et al. (2016-06-15). “GW151226: Observation of Gravitational Waves from a 22-Solar-Mass Binary Black Hole Coalescence”. Physical Review Letters. 116 (24): 241103. doi:10.1103/PhysRevLett.116.241103. PMID 27367379. ↩
- S. Kolkowitz et al. “Gravitational wave detection with optical lattice atomic clocks” Phusical Review D 94, 124043 (2016) doi: 10.1103/PhysRevD.94.124043 ↩
1 comment
[…] En vez de usar láseres para detectar ondas gravitacionales como en LIGO podrían usarse relojes atómicos. Víctor Marin en The gravitational wave detector revisited: a new approach using atomic clocks […]