The Spanish take on the nature of the neutrino: the NEXT Experiment
In our current understanding of the nature there still are some big gaps of knowledge that reminds us of the long journey ahead in physics. Here I will be talking about one of the great mysteries of our time in science, and the great endeavor taken by some of the most skilled people I’ve ever known: the nature of the neutrino and the NEXT experiment. Deep below the Spanish side of the Pyrenees one can find the LSC (Laboratorio Subterráneo de Canfranc – Canfranc Underground Laboratory) where some of the most advanced experimental research in particle physics – in Spain – takes place. There is based the NEXT experiment, a particle detector able to catch one of the remaining holy grails of particle physics: the neutrinoless double beta decay. A disintegration that, if detected (never has been, that’s the whole purpose of NEXT), would prove that the neutrino is its own antiparticle, a result with profound meaning not only in the nature of the neutrino itself, but also in the creation of our universe.
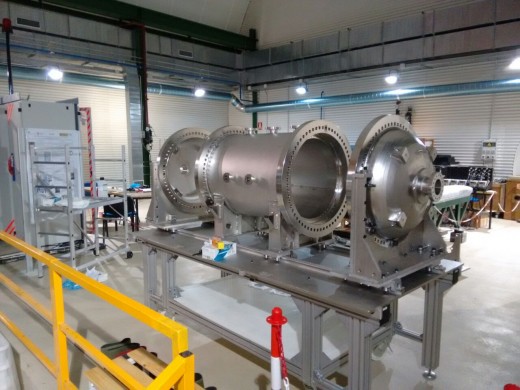
Historically we should go back to the Italian physicist Ettore Majorana. Born in Sicily in 1906 we are talking about one of the greatest geniuses in our recent history. With a mysterious and full of troubles life (and I totally recommend you reading his biography), he made major contributions to theorethical physics and, in particular, to the nature of the neutrino. One of his most interesting ideas was that, maybe, the neutrino could be its own antiparticle 1, a Majorana Fermion, in opposition to a Dirac fermion, particles that are not their own antiparticles (most of the ordinary matter). With the exception of the neutrino all the Standard model fermions are Dirac fermions, while the nature of the neutrino is not settled yet. Concluding that the neutrino is a Majorana particle would be really interesting because that would allow a process called leptogenesis, the non-conservation of a magnitude called the leptonic number, one of the key characteristics in a fermion. And that would also be really interesting because there would be an explanation to the matter-anti matter asymmetry: the creation of slightly different amounts of matter and antimatter in the big bang that, once annihilated, leaved a small amount of matter intact generating all our observable universe. Why there was slightly more matter created at the beggining? So yes, that would answer one of the biggest questions in modern cosmology. How would NEXT do it?
It is clear that NEXT has to detect something implying a Majorana neutrino. We are lucky since the radioactive isotope 136 of Xenon can undergo a special kind of decay known as double beta decay. In that event, two electrons and two neutrinos are emitted from the Xenon atom. One can think that, if the neutrino is its own antiparticle, when putting two neutrinos in the same spot they will annihilate. If we have a double beta decay, then, there should be a chance that the neutrinos annihilate themselves and only electrons are observed. The actual mechanism is rather complex, this is just a simplification to make things more understandable, but at the end, if the neutrino is a Majorana particle, a double beta decay could only emit two electrons and not the whole pack of electrons and neutrinos. NEXT aims to detect a neutrinoless double beta decay, then.
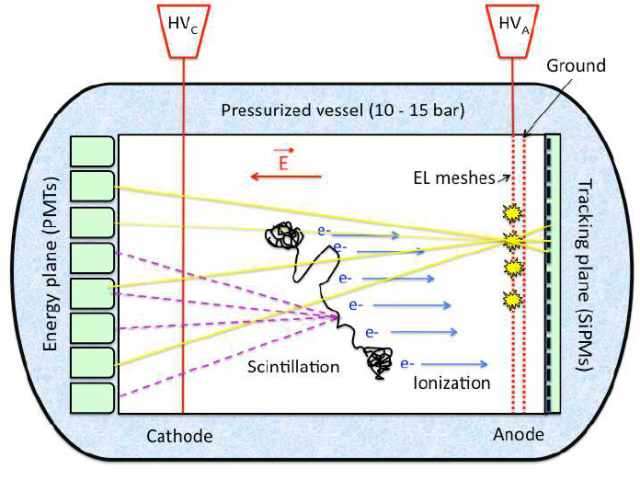
But, how are going to do it? They are building a beautiful piece of craftsmanship with a clockwork-like detection mechanism: a time projection chamber. The idea is simple: let’s put a lot of Xenon in a pressure vessel, let’s fill the vessel surfaces with detectors, and then let’s wait to a neutrinoless double beta decay to happen. Miles away from easy in the sensing side indeed. They want to detect the double beta event with two different mechanisms, just to be sure. The first is to measure the energy of the event. Neutrinos only interact via weak force with matter, meaning that usually they just cut trough anything without them even noticing, so they cannot measure the energy of a neutrino if they are emitted. But electrons are quite easy to see. Xenon is also helping: it is a scintillating material. It shines when something charged travels through it. An electron at great speeds would rip apart some of the electrons in the Xenon atoms, emitting a birght light pulse in the process. The amplitude of that pulse is proportional to the energy of the electrons and NEXT can use it to measure the energy of the decay. If a decay takes place involving two electrons and two neutrinos all the energy will be divided between the four particles, meaning that the sum of the energies of the electrons (the thing that NEXT can detect) never will be equal to the energy of the decay itself. It there are zero neutrinos emitted (confirming the Majorana hypothesis) all the energy of the decay will go to the electrons then. So, measuring that scintillation light one can tell the difference between a normal double beta from a neutrinoless double beta decay. But, there is also another important trait of the event: its topology. A neutrinoless double beta event would produce two opposing electron traces coming from the very same spot, ending in energy blobs (because the slower an electron travels in Xenon, the higher its energy deposition), so measuring the event shape one can also tell a neutrinoless double beta event from anything else. To achieve that an electrical field drifts the excited Xenon atoms, now charged because the electrons from the double beta ionized them, towards the end of the vessel. There another much intense electrical field takes over, accelerating all the excited Xenon atoms, making them rip apart new Xenon atoms and, at the end, creating a big flash with the shape of the original event (a phenomenon known as electroluminiscence). Right after that there is a pixel matrix that captures that flash, reconstructing the shape and energy, again, of the original event. So, double confirmation!
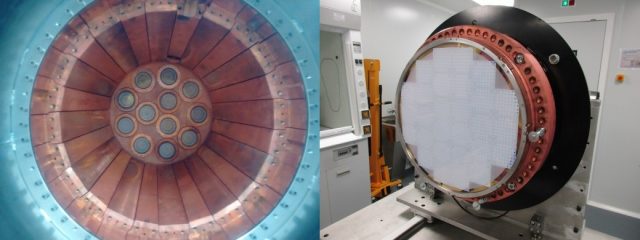
Its always very important to double check the result, specially in this experiment. I’ve worked there as an intern student for two years. There I dealt with a technology demonstrator known as NEXT-DEMO, a downscaled version of the full experiment. There we turned on the detector, measure the environment and some known radioactive samples to calibrate the sensors, and run some analysis. We gathered a million of events (coming from all kinds of sources), in the downscaled version of the experiment in a matter of days. So there was a lot of background to deal with. And, if the neutrino is a Majorana particle, in the full scale one ton of Xenon NEXT one could expect about one event per year. One neutrinoless double beta decay per year. Just one. And they have to catch it. They have to see it in a sea of hundreds of millions of events coming from other sources. Yes, they have to double check what are they seeing. In fact, that particular design allows to reject most of the background. Almost nothing should have the energy and shape of a neutrinoless double beta decay apart from an actual one. They are also very concerned with the energy resolution, meaning, how precisely can they measure the energy of the event, with good reason. There is a natural source that emits at a very very similar energy to the double beta one, so they need a really good resolution to tell them apart regarding energy measurement. They’ve achieved a fantastic resolution lower than a 1% of the energy of the decay (2458.1 KeV), allowing them to deal with that problem. Also, with the latest update in the detector characterization they concluded that the final detector will reach the required sensitivity to the decay after just three operational years 2. So, cheers, mates! Let’s go for it! Worldwide recognition awaits!
References
- Majorana, Ettore; Maiani, Luciano (2006). “A symmetric theory of electrons and positrons”. In Bassani, Giuseppe Franco. Ettore Majorana Scientific Papers. pp. 201–33. doi:10.1007/978-3-540-48095-2_10. ↩
- NEXT Collaboration (J. Martín-Albo et al.) (2016) Sensitivity of NEXT-100 to neutrinoless double beta decay JHEP DOI: 10.1007/JHEP05(2016)159 ↩
2 comments
[…] Neutrinoen izaerari buruzko esperimenturik interesgarrienetariko bat Espainian egiten ari dira. Víctor Marínek aurkezten digu: The Spanish take on the nature of the neutrino: the NEXT Experiment. […]
[…] Uno de los experimentos más interesantes sobre la naturaleza de los neutrinos se lleva a cabo en España. Víctor Marín en The Spanish take on the nature of the neutrino: the NEXT Experiment […]