Attoscience using X-ray free-electron lasers
How long does it take a photon to split a molecule? It is apparent that the answer must be well bellow a second, but, how much bellow? Attoscience focuses on these kind of phenomena that occur at the attosecond time-scale using pulses of electrons or photons. The prefix ‘atto-‘, symbol a, means 10-18, hence an attosecond is 10-18 seconds.
High harmonics generation is almost a prerequisite for attophysics. This is a non-linear process during which a target is illuminated by an intense laser pulse; the sample will emit as a response the high harmonics of the generation beam. Attosecond science based on high-harmonic generation of optical lasers has led to new insights into fundamental quantum-mechanical dynamics. It provides previously inaccessible knowledge and control of processes such as atomic electron tunnelling and photoemission delay, transport delay times in solids, coherent electron dynamics in both atoms and molecules, proton dynamics in molecules and light-field-controlled reversible phase transitions in dielectrics.
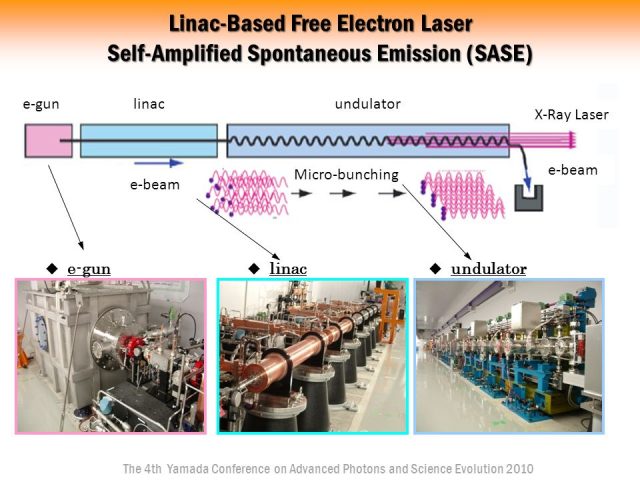
Enter the free-electron laser. This type of laser works by the stimulated emission of electromagnetic radiation produced by the motion of electrons moving rapidly in external magnetic fields. A beam of electrons at relativistic speeds is produced in an accelerator and passed into a region in which the electrons are forced to follow a sinusoidal path by an array of magnets – the so-called ‘undulator’. The associated synchrotron radiation is highly coherent as in a conventional laser. Free-electron lasers can be tuned to a narrow frequency range and radiation can be produced over a wide range, from microwaves to soft X-rays.
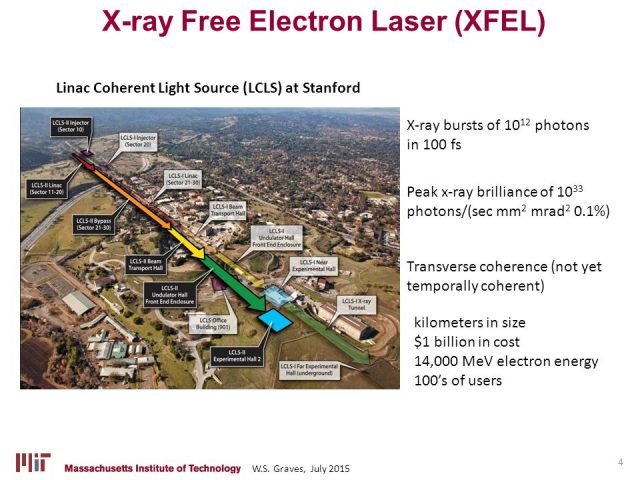
Novel ultrabright X-ray free-electron laser (XFEL) facilities have been developed, which have opened the door to high-intensity X-ray experiments in the physical, chemical, life and material sciences, with implications to state-of-the-art technology and modern medicine. However, in spite of pioneering results of coherent control with seeded free-electron lasers, most of these new XFEL sources still rely on the principle of self-amplification of spontaneous emission (SASE) by relativistic electrons in the periodically varying magnetic field of an undulator. The consequence is that they produce ultrashort bursts of X-rays with energies of up to tens of millijoules at wavelengths down to the ångström level, corresponding to approximately 1012 photons per pulse in the photon energy range from 0.5 keV to 8 keV. Thus, XFELs extend ultrafast science to the X-ray regime, complementing state-of-the-art high harmonics generation sources.
Or so it was expected, because combining attosecond temporal resolution with high-brilliance sub-nanometre-scale coherent X-rays at XFELs has eluded researchers, largely due to the stochastic nature of their generation, but also because a lack of knowledge about the temporal structure and instantaneous frequency of individual SASE pulses.
Now, an international team, incluiding Ikerbasque Research Professor at UPV/EHU & DIPC Andrey Kazansky, report 1 the measurement of attosecond time–energy information of individual XFEL pulses. The measurement is done through angular streaking of X-ray-generated photoelectrons with a circularly polarized infrared (IR) laser pulse, where the time axis is mapped onto the angular axis. From this, the team reconstructs the streaking field amplitude and phase as well as the intensity structure and chirp of the XFEL pulses on a single-shot basis with attosecond resolution. The results not only confirm fundamental aspects of X-ray pulse generation in XFELs, they also allow for sophisticated sorting of experimental data.
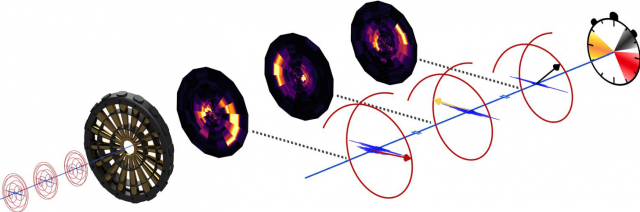
The time–energy information of ultrashort X-ray free-electron laser pulses generated by the Linac Coherent Light Source is measured with attosecond resolution via angular streaking of neon 1s photoelectrons. The X-ray pulses promote electrons from the neon core level into an ionization continuum, where they are dressed with the electric field of a circularly polarized infrared laser. The reasearchers found that the reconstructed substructure of the XFEL pulses is consistent with lower-resolution, indirect X band transverse cavity (XTCAV) measurements as well as with theoretical predictions of the SASE process.
Demonstrated for soft X-rays, this measurement also scales to hard X-rays, with their accompanying even shorter SASE spike durations. Changing the target gas, and hence the absorption edges to the required higher X-ray energy range, while at the same time decreasing the streaking wavelength allows a straightforward adaptation of the set-up to the specific needs of hard X-ray pulse characterization.
Together with recent proposals for attosecond X-ray pulse generation at XFELs in combination with new XFEL pulse-shaping techniques and synchronized IR lasers, this brings the full arsenal of lab-based attoscience to the high-intensity regime of XFELs.
Author: César Tomé López is a science writer and the editor of Mapping Ignorance.
References
- N. Hartmann, G. Hartmann, R. Heider, M. S. Wagner, M. Ilchen, J. Buck, A. O. Lindahl, C. Benko, J. Grünert, J. Krzywinski, J. Liu, A. A. Lutman, A. Marinelli, T. Maxwell, A. A. Miahnahri, S. P. Moeller, M. Planas, J. Robinson, A. K. Kazansky, N. M. Kabachnik, J. Viefhaus, T. Feurer, R. Kienberger, R. N. Coffee & W. Helml (2018) Attosecond time–energy structure of X-ray free-electron laser pulses Nature Photonics doi: 10.1038/s41566-018-0107-6 ↩