How to (potentially) repair our brain using our wisdom teeth
Authors: Beatriz Pardo-Rodríguez, Gaskon Ibarretxe and José R. Pineda. Department of Cell Biology and Histology. Faculty of Medicine and Nursery of the University of the Basque Country (UPV/EHU).
One of the most famous quotes that Santiago Ramón y Cajal wrote in his work “Rules and tips on scientific research” was “Every man can, if he so desires, become the sculptor of his own brain.” 1. However, the use of dental material as mud and stones to model or repair sculptures probably sounded like science fiction at the time…
The human central nervous system (CNS) has a very limited capacity for self-repair. Indeed, the amelioration after traumatic brain injuries or neurodegenerative diseases is challenging, focusing on protecting and stimulating the plasticity (reconnecting wires) of the remaining living neuronal cells. For this reason, traditional treatments often focus on symptom management rather than tissue regeneration. In the field of cell therapy, stem cells offer an undeniable advantage, given that they are mouldable: their function is to be a cellular reservoir with well-cared for and unpolluted genetic material, ready to activate the cell to be integrated into a damaged environment. In theory, stem cell therapy presents a promising avenue for CNS repair; however, the current drawbacks are 1) The CNS constitutes a very particular environment where only the essentials are found in a perfectly organized manner, and any additional element is a hindrance and 2) the sourcing of appropriate stem cells remains a hurdle, as it is well-known that normal stem cells can sometimes transform into cancer-stem cells may contribute to a life-threatening tumorigenesis on the grafted organ. Indeed, multiple tumours in which the presence of cancer stem cells has been identified include brain, breast, liver, pancreas, colorectal, lung, stomach, prostate, skin, liver, ovarian, bone even myeloid leukemia 23456789.
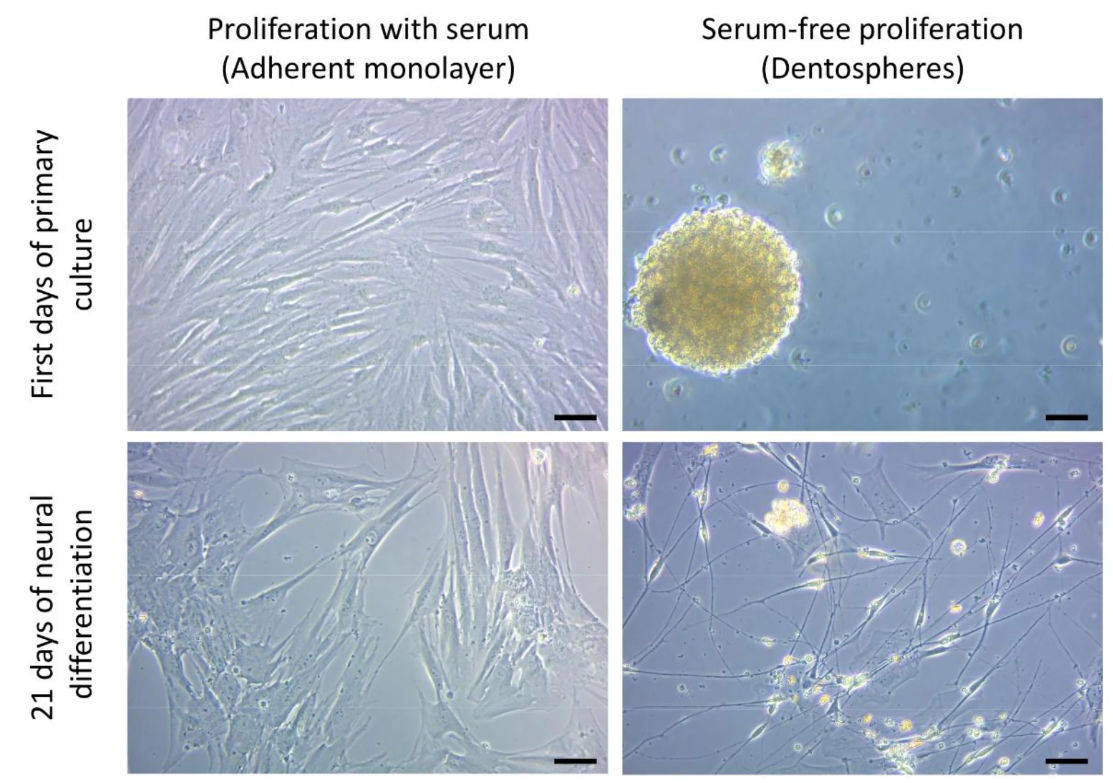
Interestingly, human dental pulp stem cells (hDPSCs) are a special type of stem cell derived from the dental pulp of extracted teeth, of which no tumorigenic capacity has been reported to date 10. Furthermore, the non-requirement of intrusive genetic modification to differentiate them toward a post-mitotic neuronal phenotype endows them with additional safety guarantees 11. Another important advantage of their use is that they offer an accessible and ethically uncompromised cell source with the potential to differentiate into various cell types and secrete neuroprotective factors which are beneficial for neural survival and growth.
The biggest challenge is finding a way to create a functional neuronal cell from a human adult non-CNS derived stem cell such as hDPSCs. In research, work is always an evolution of previous work. In 2004, 21 years ago, researchers at the University of Barcelona succeeded in differentiating neurons from embryonic precursors of rat striatal cells, capable of integrating into damaged brains 12. In the current work of Pardo-Rodriguez and collaborators, based on modifications of the previously published protocol, they found the solution to integrate the requirements of adult human stem cells culture and the proper signals and cues to generate functional neurons from hDPSCs 13.
The primary objective of the study was to improve existing protocols for hDPSCs culture. The presence of serum in culture media (10% fetal bovine serum, or FBS) usually helps the cells to survive and grow. However, sustained FBS presence over time restricts the potential for stem cell differentiation. Cell differentiation is like a journey in which the direction must be carefully decided, since one cannot go to all cardinal points at once. The study assessed the impact of serum presence during the initial expansion phase on subsequent neural differentiation. Serum-free culture media more specific for Neural Stem Cells were selected, allowing the generation of spheroid cultures (dentospheres) similar to neurospheres. The results showed that hDPSCs cultured initially in serum-free conditions (spheroids) exhibited a higher propensity for neural differentiation compared to those cultured with FBS (Figure 1).
Next, the differentiation protocol was enhanced by incorporating retinoic acid (RA) and potassium chloride (KCl) pulses. After the initial culture phase, cells were subjected to a neural induction medium for 21 or 60 days, supplemented with RA and KCl to promote neuronal differentiation. They characterized the molecular and morphological characteristics of the differentiated cells through the reverse transcription quantitative polymerase chain reaction (RT-qPCR, used to quantify gene expression levels of neural markers and ion channel subunits) and immunofluorescence technique (this technique allows to specifically detect the presence of particular cell components, such as in this case neural markers). Moreover, they performed a digital morphometric analysis through 3D-Sholl (this is a particular image analysis for assessing cell morphology and complexity of neurite outgrowth (defining the neuronal shape)). The results showed that serum-free cultures increased the expression of neural progenitor markers such as Nestin, GFAP, S100β, and p75NTR. After hDPSCs differentiation, cells expressed neuronal markers including doublecortin (DCX), neuronal nuclear antigen (NeuN), Ankyrin-G, and microtubule-associated protein 2 (MAP2) together with the presence of presynaptic proteins (vGLUT2, Synapsin-I) and postsynaptic scaffold proteins associated with both excitatory (glutamatergic) and inhibitory (GABAergic) synapses suggesting their potential to form synaptic structures.
Finally, the functional characteristics of the obtained cells were assessed through electrophysiological analysis using whole-cell patch-clamp recordings (this technique evaluates the functional electrical properties of the differentiated cells). The results showed that differentiated hDPSCs had voltage-dependent potassium (K⁺) and tetrodotoxin (TTX)-sensitive sodium (Na⁺) currents, which are essential for action potential generation. Moreover, the generated cells exhibited spontaneous electrophysiological activity and could generate repetitive action potentials with full baseline potential recovery, demonstrating functional excitability akin to neurons. The conclusion is that the treatment with RA and KCl led to an upregulation of voltage-gated Na⁺ and K⁺ channel subunits at the transcript and protein level, thus correlating with the observed electrophysiological properties.
In conclusion, the culture of hDPSCs in serum-free conditions prior to differentiation enhanced the neural differentiation capacity of hDPSCs and the incorporation of RA and KCl in the differentiation protocol effectively promoted a successful maturation process, where cells generated electrical impulses characteristic of mature neurons. This study demonstrates that hDPSCs can be effectively differentiated into neuron-like cells exhibiting functional electrophysiological activity, without extrinsic genetic modification.
The successful generation of functional neuron-like cells from hDPSCs opens avenues for creating in vitro models for neurodegenerative diseases and drug screening. New autologous transplantation strategies could be developed to replace lost or damaged neurons in patients with CNS injuries or degenerative conditions. It is hoped that this study will foster new advancements in the field of regenerative medicine, by providing a reliable and ethical source of functional neural cells for future translational research applications.
As Cajal said, the ideas do not last unless one has the determination and the courage to bring them to execution.
References
- Ramón y Cajal, Santiago (2016). Reglas y consejos sobre investigación científica. Los tónicos de la voluntad (en español). Gadir. ISBN 9788494576584. ↩
- Sheila K Singh, Cynthia Hawkins, Ian D Clarke, Jeremy A Squire, Jane Bayani, Takuichiro Hide, R Mark Henkelman, Michael D Cusimano, Peter B Dirks. Identification of human brain tumour initiating cells. Nature. 2004 Nov 18;432(7015):396-401. doi: 10.1038/nature03128. ↩
- Muhammad Al-Hajj, Max S Wicha, Adalberto Benito-Hernandez, Sean J Morrison, Michael F Clarke. Prospective identification of tumorigenic breast cancer cells. Proc Natl Acad Sci U S A. 2003 Apr 1;100(7):3983-8. doi: 10.1073/pnas.0530291100. ↩
- A Eramo, F Lotti, G Sette, E Pilozzi, M Biffoni, A Di Virgilio, C Conticello, L Ruco, C Peschle, R De Maria. Identification and expansion of the tumorigenic lung cancer stem cell population. Cell Death Differ. 2008 Mar;15(3):504-14. doi: 10.1038/sj.cdd.4402283. ↩
- Collins AT, Berry PA, Hyde C, Stower MJ, Maitland NJ. Prospective identification of tumorigenic prostate cancer stem cells. Cancer Res. 2005 Dec 1;65(23):10946-51. doi: 10.1158/0008-5472.CAN-05-2018. ↩
- Yamashita T, Ji J, Budhu A, Forgues M, Yang W, Wang HY, Jia H, Ye Q, Qin LX, Wauthier E, Reid LM, Minato H, Honda M, Kaneko S, Tang ZY, Wang XW. EpCAM-positive hepatocellular carcinoma cells are tumor-initiating cells with stem/progenitor cell features. Gastroenterology. 2009 Mar;136(3):1012-24. doi: 10.1053/j.gastro.2008.12.004. ↩
- D Bonnet, J E Dick. Human acute myeloid leukemia is organized as a hierarchy that originates from a primitive hematopoietic cell. Nat Med. 1997 Jul;3(7):730-7. doi: 10.1038/nm0797-730. ↩
- Jamieson CH, Ailles LE, Dylla SJ, Muijtjens M, Jones C, Zehnder JL, Gotlib J, Li K, Manz MG, Keating A, Sawyers CL, Weissman IL. Granulocyte-macrophage progenitors as candidate leukemic stem cells in blast-crisis CML. N Engl J Med. 2004 Aug 12;351(7):657-67. doi: 10.1056/NEJMoa040258. ↩
- Pineda JR, Badiola I, Ibarretxe G. Stem and Cancer Stem Cell Identities, Cellular Markers, Niche Environment and Response to Treatments to Unravel New Therapeutic Targets. Biology (Basel). 2021 Jan 2;10(1):25. doi: 10.3390/biology10010025. ↩
- Crende O, García-Gallastegui P, Luzuriaga J, Badiola I, de la Hoz C, Unda F, Ibarretxe G, Pineda JR. Is There Such a Thing as a Genuine Cancer Stem Cell Marker? Perspectives from the Gut, the Brain and the Dental Pulp. Biology (Basel). 2020 Nov 27;9(12):426. doi: 10.3390/biology9120426. ↩
- Luzuriaga J, Polo Y, Pastor-Alonso O, Pardo-Rodríguez B, Larrañaga A, Unda F, Sarasua JR, Pineda JR, Ibarretxe G. Advances and Perspectives in Dental Pulp Stem Cell Based Neuroregeneration Therapies. Int J Mol Sci. 2021 Mar 29;22(7):3546. doi: 10.3390/ijms22073546. ↩
- Bosch M, Pineda JR, Suñol C, Petriz J, Cattaneo E, Alberch J, Canals JM. Induction of GABAergic phenotype in a neural stem cell line for transplantation in an excitotoxic model of Huntington’s disease. Exp Neurol. 2004 Nov;190(1):42-58. doi: 10.1016/j.expneurol.2004.06.027. ↩
- Pardo-Rodríguez B, Baraibar AM, Manero-Roig I, Luzuriaga J, Salvador-Moya J, Polo Y, Basanta-Torres R, Unda F, Mato S, Ibarretxe G, Pineda JR. Functional differentiation of human dental pulp stem cells into neuron-like cells exhibiting electrophysiological activity. Stem Cell Res Ther. 2025 Jan 23;16(1):10. doi: 10.1186/s13287-025-04134-7. ↩