Geological phenomena implying dissolved species bring new insights on fundamental thermophysics
Over geologic time scales, seawater transforms the basalt of the ocean floor by chemical attack. At the end of this alteration process, the basalt turns partly into clays and partly into dissolved salts in sea water. Lithium, a chemical element initially contained in basalt, will then be distributed between clay and seawater. This separation has important consequences on the properties of lithium.
In nature, lithium atoms can be of two varieties, called “isotopes”. These two forms, denoted 7Li and 6Li, have different masses (7 and 6) and will have different affinities for clay and sea water. Specifically, the 7Li isotope is found to be more concentrated in the solution than in the clay. In the same way, most of the geological processes shaping our planet lead to isotopic enrichments that are specific to them, and which in a way constitute their signature. The 7Li concentration measured in the clay can then be used (see Figure 1.) to reconstruct the history of the geological events this clay has known, such as the chemical attack of basalt. This concentration can be used to reconstruct past climates or, in other cases, to understand the internal dynamics of the Earth or the formation of the solar system.
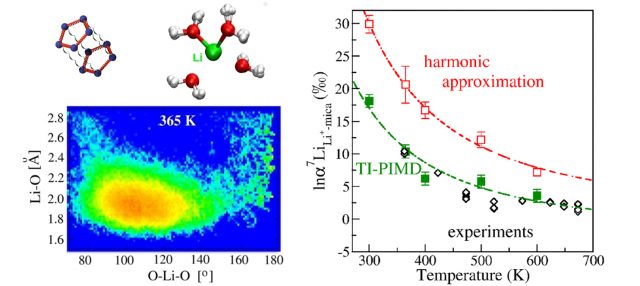
In order to use these concentration measurements, it is, however, necessary to understand fully these enrichment mechanisms. For this purpose, laboratory experiments can be carried out, reproducing as closely as possible the natural conditions. However, as these experiments are expensive and time-consuming, an alternative and simpler approach is to deduce these enrichment properties from theoretical calculations based on the modeling of quantum phenomena taking place at the atomic scale. At this scale, the atoms are attached in pairs by bonds, such as lithium with the oxygen atom of the surrounding water molecules (see figure 1). These bonds act somewhat as a spring or an elastic between the two atoms, which begin to vibrate under the effect of molecular agitation. The 7Li’s preference for sea water then comes from its larger mass, which will make it vibrate with oxygen more slowly than 6Li. To understand this preference, one must understand the dynamical behavior of the lithium atom and how it differs in seawater and clay.
A very effective approximate method, called “harmonic approximation”, assumes a proportionality between the elongation of the bond and its retraction force. This approximation was developed 60 years ago and has been applied to estimate these dynamical and isotopic enrichment properties for many solid species, such as the minerals constituting rocks, and gaseous species, such as the molecules constituting the atmosphere. Historically, the harmonic approximation has been the starting point for our understanding of the effects of isotopic enrichment, however its application to liquids has long been problematic. Liquids, although of great importance in natural processes, are, on the one hand, more difficult to study experimentally for their dynamic properties, and on the other, they exhibit complex dynamic behavior, which could make this approximate approach fail.
In this work 1 , focusing on the specific case of lithium, we show undoubtedly that for liquids, another approach must be used. This approach, called “path integral molecular dynamics”, is based upon the formulation of quantum mechanics developed in the 1940s by Richard Feynman, 1965 Nobel Prize in Physics, and allows the computation of isotopic enrichment accurately.
Applying this approach to the lithium case, we show for the first time that it is essential to go beyond the limits of the harmonic approximation in order to understand the isotopic enrichment processes of liquids.
This work thus lifts the last remaining lock to our theoretical understanding of the mechanisms of isotopic fractionation, covering the case of liquids. Importantly, path integral methods, being fully atomistic, allow us to identify the origins of anharmonic effects and to make reliable predictions at temperatures that are experimentally inaccessible yet are, nevertheless, relevant for natural phenomena.
Author: Romain Dupuis is a young scientist who earned his Ph.D. in 2014 at the Université Paul Sabatier Toulouse III in France. Since then, he has been working at the Donostia International Physics Center as a postdoctoral fellow studying thermodynamical properties of cement-based materials. His research is dedicated to the simulation of solids and liquids at the atomic scale by the means of molecular dynamics. He is strongly interested in isotopic fractionation and its relation to the structural properties of matter.
References
- Romain Dupuis, Magali Benoît, Mark E. Tuckerman, and Merlin Méheut (2017) Importance of a fully anharmonic treatment of equilibrium isotope fractionation of dissolved ionic species as evidenced by li+(aq) Acc. Chem. Res. doi: 10.1021/acs.accounts.6b00607 ↩