Is your skin under fracking?
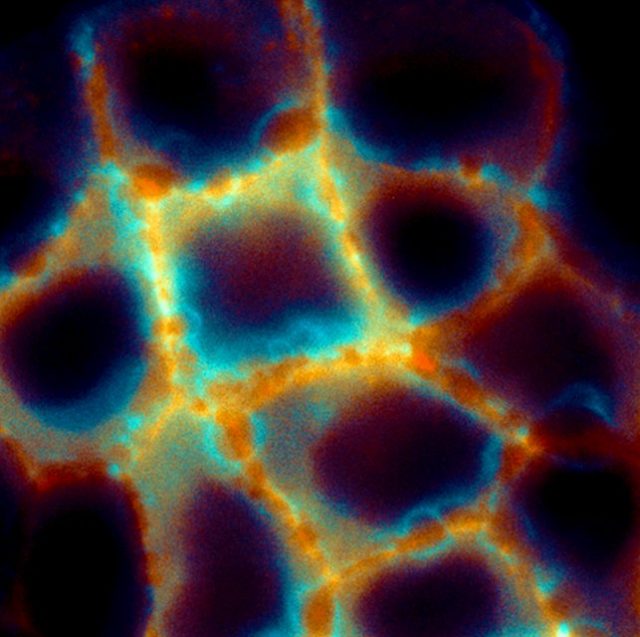
Hydraulic fracturing or Fracking is a controversial technique in which a solvent (water with chemicals) is injected at high pressure into deep rocks in order to break them so that natural gas and petroleum can flow freely.
In a paper published recently in Nature Materials1, researchers from the Institute of Bioengineering of Catalonia claim that epithelial cells in our body may fracture under the exact same mechanism. Don’t panic! The consequences in this case are different. The epithelium, contrary to the soil, is a living material capable of repairing the damage.
Epithelial cells and the extracellular matrix
Epithelial cells cover many surfaces and internal cavities in our body. Like cells from our skin, their main function is to shape a physical barrier that protects us from pathogens, and physical damage. For this reason, epithelial cells are tightly connected to each other, holding hands to maintain the cohesion of the tissue. And they are also adhered to a soft substrate that is called the extracellular matrix. The extracellular matrix is a porous polymer matrix made of fibrous proteins such as collagen or Matrigel (which you may have heard about in skin product advertisements) and filled in with solvent.
But epithelial tissues are not static. Under normal conditions, most cells and organs in our body are continuously under stretch: our lungs expand and relax every time we breath; our heart and vessels are continuously under deformations due to blood pressure; the breasts and the skin are highly stretched during pregnancy and lactation; the oesophagus, the stomach and the intestines are affected by peristaltic contractions generally associated with digestion; our muscles deform every time we move; etc. Tissue failure to withstand these deformations could lead to pathological conditions2.
The work
In this work, researchers set up to understand how the epithelium fractures under deformations, specifically under stretch-unstretch manoeuvres. Previous studies have shown that epithelial fracture is caused by excessive tension in the tissue34. In this paper, however, a novel mechanism for epithelial fracture is found which is not tensile but hydraulic.
The experimental setup consists of a stretching device that allows them to stretch groups of epithelial cells adhered on an extracellular matrix and image the cells under the microscope during the process. With this stretching device they try to assess how the epithelium resists tension and how it fractures. Surprisingly, as they stretch up to 15%, they cannot break the tissue. However, it iswhen they release this stretch that the epithelium fractures. Sub-cellular cracks appear between cells and heal after a few minutes. This finding is shocking. How can the epithelium resist stretch but fail under compression? Besides, after stretch release tension in the tissue drops dramatically, and yet it is at this point that the tissue fractures. Then, if it is not tension, what is the mechanism underneath this fracture?
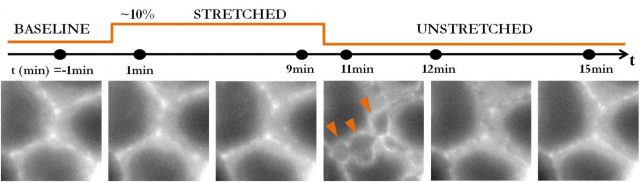
The key experiment that gives us the clue about the mechanism at play is when researchers stretch cells adhered on silicon membranes without extracellular matrix. Then, cells do not fracture: not during, not after stretch release. Therefore, it is clear that the extracellular matrix is playing a role in this kind of fracture. And this role is given by its hydrogel nature.
The best way to understand this mechanism is to think of the extracellular matrix as a sponge inmersed in fluid. When a sponge is compressed, it releases water; when a sponge is stretched, it swells absorbing water. Similarly, hydrogels like the extracellular matrix swell during stretch and release water when compressed. This water released by the extracellular matrix, in its way out, hits the basal part of the cells creating epithelial cracks that heal when the flux of water decreases. In this paper, researchers use the Theory of Poroelasticity to describe the behaviour of the extracellular matrix. They develop a model based on this theory that successfully captures the behaviour of hydrogel substrates. But what is more interesting is that with this model they are also able to predict the size and healing dynamics of the epithelial cracks as a function of the stiffness, geometry and composition of the hydrogel. For example, in a thick hydrogel the solvent will need more time to equilibrate and therefore epithelial cracks will remain open longer times. Similarly, in soft hydrogel the pressure of the fluid will be lower and therefore the amount of epithelial fracture is reduced.
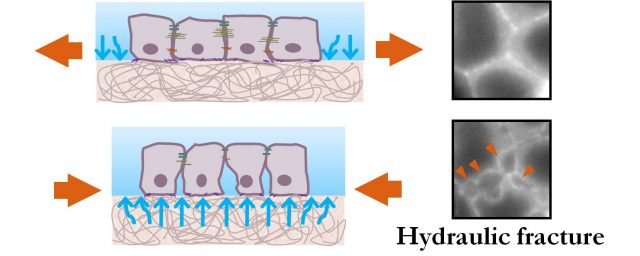
Overall, in this study, researchers put forward a new type of mechanism for epithelial tissues under stretch – hydraulic fracture – whose principle is already familiar in the fracking industry.
Implications and future research
This study sets new concerns and limitations in the fields of biomaterials and tissue engineering. Do prospective materials for implants and tissue scaffolds generate poroelastic flows under deformation? Do these flows allow for optimal tissue regeneration? Because this hydraulic fracture applies both to synthetic and physiological hydrogel substrates, poroelastic flows should be taken into account when designing new hydrogel biomaterials for implants.
On the other hand, hydraulic fracture provides a new strategy for drug delivery. Hydraulic fractures open transient fractures in tissues, creating new paths by which poroelastic flows could reach otherwise inaccessible locations and deliver pharmaceutical compounds in a controlled manner.
References
- Casares L., Dobryna Zalvidea, Noelia Campillo, Daniel Navajas, Marino Arroyo & Xavier Trepat (2015). Hydraulic fracture during epithelial stretching, Nature Materials, 14 (3) 343-351. DOI: http://dx.doi.org/10.1038/nmat4206 ↩
- Suki, B. & Hubmayr, R. (2014) Epithelial and endothelial damage induced by mechanical ventilation modes. Curr Opin Crit Care 20, 17-24, doi: 10.1097/MCC.0000000000000043 ↩
- Trepat, X. et al. (2004) Viscoelasticity of human alveolar epithelial cells subjected to stretch. American journal of physiology287, L1025-1034, doi: 10.1152/ajplung.00077.2004 ↩
- Harris, A. R. et al. (2012) Characterizing the mechanics of cultured cell monolayers. Proc Natl Acad Sci U S A109, 16449-16454, doi:10.1073/pnas.1213301109 ↩