An acoustic quantum bus
At a fundamental level the term “quantum computing” implies de use of quantum effects that have no classical analogue to process information. In a “classical computer” information is held in bits, which can have two alternative values (0 and 1). quantum computer the values 0 and 1 are held simultaneously in an entangled state. This unit of information is a quantum bit (qubit). Much more information can be held in this way and, in principle, it is possible to do parallel processing of the information. Quantum computers would be much faster than conventional machines and capable of performing calculations that could not realistically be done otherwise. In research some physical systems has been used to substantiate quantum computers, like ion traps or spin measurements.
But qubits need to interact and long-range interactions between remote qubits are arguably one of the greatest challenges towards developing a scalable, solid-state spin-based quantum information processor. One approach to address this problem is to interface qubits with a common quantum bus that distributes quantum information between distant qubits.
The transduction of quantum information between stationary and moving qubits is central to this approach. A particularly efficient implementation of such a quantum bus can be found in the field of circuit quantum electrodynamics where spatially separated superconducting qubits interact via microwave photons confined in transmission line cavities. In this way, multiple qubits have been coupled successfully over relatively large distances of the order of millimetres. Fueled by dramatic advances in the fabrication and manipulation of nanomechanical systems, an alternate line of research has pursued the idea of coherent, long range interactions between individual qubits mediated by mechanical resonators, with resonant phonons, quanta of crystal lattice vibrational energy, playing the role of cavity photons.
Now an international group of researchers that includes Geza Giedke, an Ikerbaske Research Fellow working at DIPC, and led by Juan Ignacio Cirac, of the Max Planck Institute for Quantum Optics, propose1 a new quantum transducer and data bus based on surface acoustic waves (SAWs).
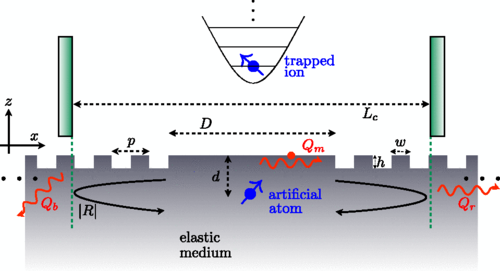
SAWs involve phonon-like excitations bound to the surface of a solid and are widely used in modern electronic devices, e.g., as compact microwave filters. The researchers propose and analyze SAW phonon modes in piezoactive materials as a universal mediator for long range couplings between remote qubits.
An interesting point of the new transducer is that it is not specific to any particular qubit realization, but—thanks to the variety of physical properties associated with SAWs in piezoactive materials (strain and electric and magnetic fields)—provides a common on-chip platform accessible to various different implementations of qubits, comprising both natural (e.g., ions) and artificial candidates, such as quantum dots or superconducting qubits. In particular, this opens up the possibility to interconnect dissimilar systems in new electroacoustic quantum devices.
Most importantly, the new transducer is built upon an established technology: Lithographic fabrication techniques provide almost arbitrary geometries with high precision as evidenced by a large range of SAW devices such as delay lines, bandpass filters, resonators, etc. In particular, the essential building blocks needed to interface qubits with SAW phonons have already been fabricated, according to design principles familiar from electromagnetic devices. Not only that, the typical SAW frequencies lie in the gigahertz range, closely matching transition frequencies of quantum dots and enabling ground-state cooling by conventional and well-known cryogenic techniques.
Another important feature is that, for a given frequency in the gigahertz range, due to the slow speed of sound of ~103 m/s for typical materials, device dimensions are in the micrometer range, which is convenient for fabrication and integration with semiconductor components, and about 105 times smaller than corresponding electromagnetic resonators.
Yet another inherent advantage of the new transducer is the intrinsic nature of the coupling. In piezoelectric materials, the SAW is accompanied by an electrical potential, which has the same spatial and temporal periodicities the mechanical displacement has, and provides an intrinsic qubit-phonon coupling mechanism. This is most important because the scheme provides a new paradigm, where coupling to phonons becomes a highly valuable asset for coherent quantum control rather than a liability.
In summary, the researchers propose SAW phonons in piezoactive materials (such as GaAs) as a universal quantum transducer that allows to convert quantum information between stationary and propagating realizations. The proposed combination of techniques and concepts known from quantum optics and quantum information, in conjunction with the technological expertise for SAW devices, is likely to lead to further, rapid theoretical and experimental progress.
Once we have such a transducer, what is next? The researcers foresee some possible lines of research. First, since the transducer is not specific to any particular qubit realization, novel hybrid systems could be developed by embedding dissimilar systems such as quantum dots and superconducting qubits into a common SAW architecture. Second, the setup could also be used as a transducer between different propagating quantum systems such as phonons and photons. Light can be coupled into the SAW circuit via (for example) self-assembled quantum dots, and structures guiding both photons and SAW phonons have already been fabricated experimentally. Finally, the SAW architecture opens up a novel, on-chip test bed for investigations reminiscent of quantum optics, bringing the highly developed toolbox of quantum optics and cavity quantum electrodynamics to the widely anticipated field of quantum acoustics. Potential applications include quantum simulation of many-body dynamics, quantum state engineering (yielding, for example, squeezed states of sound), quantum-enhanced sensing, sound detection, and sound-based material analysis.
Author: César Tomé López is a science writer and the editor of Mapping Ignorance.
References
- M. J. A. Schuetz, E. M. Kessler, G. Giedke, L. M. K. Vandersypen, M. D. Lukin, and J. I. Cirac (2015) Universal Quantum Transducers Based on Surface Acoustic Waves Physical Review X DOI: 10.1103/PhysRevX.5.031031 ↩
3 comments
[…] 2016 | Computer science, Condensed matter, DIPC, Noticias, Quantum physics, Science, Technology | 0 […]
[…] An acoustic quantum bus – Mapping Ignorance https://mappingignorance.org/2016/01/21/an-acoustic-quantum-bus […]
[…] An acoustic quantum bus – Mapping Ignorance mappingignorance.org/2016/01/21/an-acoustic-quantum-bus […]