The (energetical) cost of having a brain
Our brain constitutes one of the finest pieces of natural machinery known, and it allows us to efficiently interact with our environment: searching for food, avoiding predators, communicating with other individuals, and even some more sophisticated stuff — like enjoying a well composed piece of classical (or rock) music. However, all this comes at a very high price: a considerable amount of energy. From the total of the chemical energy that we obtain from the environment in a daily basis (in form of food or breathing), our brain consumes around the 20% during its huge task of processing and interpreting the incoming information.
How does the brain consume such a large amount of energy? Most of this energy goes indeed just to maintain the brain in its “working point”, that is, to sustain the electrical charge of neurons (the membrane potential levels). However, it is also observed that active regions (in which neurons present a higher firing rate) in the cerebral cortex consume more energy than inactive ones. This is the basis of a number of brain imaging techniques (such as functional magnetic resonance imaging, or fMRI) which monitorize energy consumption to locate areas with high neural activity.

If we focus our energetical study on the computationally relevant operations, thus ignoring the part of keeping the brain in its working point, the main biophysical mechanisms which have been found responsible of large energy consumption are the generation and propagation of action potentials (the “spiky” signals generated by neurons) and the transmission of these action potentials from one neuron to another through chemical synapses, a phenomenon known as synaptic transmission. Other mechanisms, such as the ones involving the influx of calcium ions into a neuron, also use a portion of our energy, but the major part of the energy is spent in these two mechanisms (propagation of action potentials and synaptic transmission), and they have been thought during many years as roughly similar in terms of energy consumption.
However, a continuous level of controversy has been maintained during all this time, due to incompatibilities between energy-consumption estimations at the cellular level and at the level of certain non-invasive imaging methods. One of the key points in the discussion was that, at the subcellular level, the effects of certain ion fluxes may “fight” each other (for instance, one of them trying to elevate the membrane potential level while the other is trying to do exactly the opposite), thus constituting a waste of energy with no clear purpose. The clarification of such a controversy is important, for instance, for a correct interpretation of fMRI, or other useful imaging techniques available to date.
This controversy was recently smoothed by Henric Alle and colleagues 1, researchers in the Max Planck Institute for Brain Research in Frankfurt (Germany) and the Wolfson Institute for Biomedical Research, in London (UK). In their study, the researchers employed a newly developed experimental technique which allowed them to record, with a high precision and in physiologically meaningful temperatures, the ion fluxes underlying the generation of action potentials, which can be different from the ones recorded in previous studies, such as the ones in the cell body (or soma) of the neuron. They used this technique on a certain type of non-myelinated axons, the hippocampal mossy fibers, and they found that the overlap between ion fluxes with opposite effects is less prominent than what previous studies were assuming. Since the previously assumed overlap between these ion fluxes was responsible of the energy consumption estimations in the subcellular level studies, the results of Alle and colleagues indicates a lower energy consumption which fits better with the imaging-level estimations and partially solves the energy-consumption controversy in the brain.
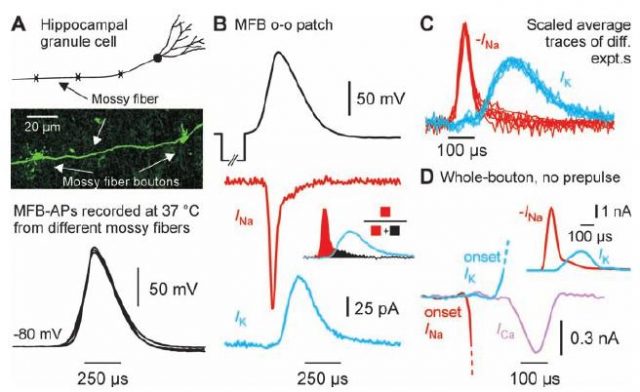
In order to obtain a more solid understanding of the phenomenon, the researchers also employed a highly detailed mathematical model to investigate the concrete effects of having a small overlap in ion fluxes. Internal currents, delays in the ion fluxes, velocity of the action potential propagation and other aspects were successfully reproduced with the model, and the low energy-consumption was replicated as well. In particular, the energy consumption was now estimated, using both the experimental and the modeling approach, in only 1.3 times the theoretical minimum of energy consumption. This is a quantity way smaller than the previous estimations for action potential propagation, which estimated the amount of consumed energy in 4 times the theoretical minimum.
These results will be helpful to better understand metabolic-based imaging technologies used to study the brain (like the fMRI mentioned before), and maybe to interpret better the resulting images which are useful for diagnostic and treatment of brain diseases. They also turn away the focus of energy-consumption from the action potential propagation to the other “big consumer” mechanism in our brain: the synaptic transmission of signals between neurons.
References
- H. Alle, A. Roth, and J. R. P. Geiger, Science 325, 1405-1408, 2009. ↩
1 comment
The problem of energy consumption in the brain neatly explained.