Sensory competition (2): A dance of electrical waves
Competition, evidence suggests, is a constant in nature. Looking from the largest to the smallest structures that Mankind has been able to observe, one can easily find examples: in clusters of galaxies (in which sometimes galaxies compete with each other to pull in the matter from their outer regions), stars (like binary systems with planetary bodies orbiting between them), or in microscopic order of certain materials, where different magnetic domains “fight each other” to reach some consensus, or macroscopic order. But of course, one of the best places to find examples of competition is in the first place we found it– Biology. From diseases like cancer (where the immune system and tumors start a years-long battle), to predator-prey situations, living organisms are a natural scenario for competition in its multiple ways. But not only individuals –unicellular or pluricellular– are the main characters in these conflicts: we can find evidences of competition in a more subtle way. For instance, different sources of information received by an animal can be seen as well as competing elements in a battle whose outcome may determine the survival of the individual.
In a previous post, I discussed the dynamics of signal competition in sensory systems, focusing on an interesting and recent example: a mechanism which explains how different odors compete in the early sensory systems of honey bees. The reader may recall that the early olfactory system of the honey bee has been found able to adapt to a particular long-present odor, and then respond energetically to a novel odor signal presented together with the old odor signal, thus allowing for an efficient discrimination of the new –and likely, more behaviorally relevant– sensory input.
It turns out that the sensory system of honey bees is not the only known neural circuit which is able to perform such a convenient discrimination. Over the last decades, there has been accumulative evidence (see 1 for a review) that weakly electric fish are able to cancel out signals related to own-body movement, while preserving signals related to the presence of prey. Such cancellation would be an efficient way to solve the competition between relevant and non-relevant signals, allowing the fish to efficiently detect and hunt the prey.
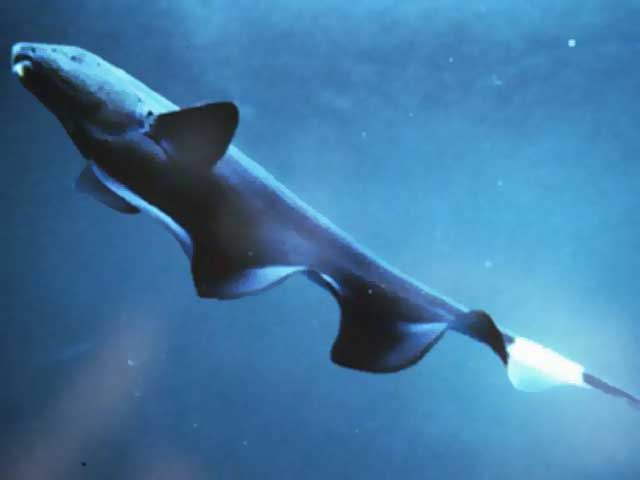
How does this cancellation work, exactly? Certain types of fish (found, for instance, in rivers across South America) are able to generate a very weak electric field that they use as a sort of radar to scout their surroundings. When some object is within a few centimeters of the fish, this object causes perturbations in the electric field, and these perturbations are detected by receptors in the body of the electric fish. This allows our fish to detect the presence of rocks, food (worms), or other fish, via the perturbation in the electric field that these objects cause. It is a very useful sensory system to use in dark, murky waters (where this fish lives), and it is known as electrolocation.
This sensory strategy has its downsides, though. The perturbations caused by prey, for instance, are usually characterized by oscillatory modulations in the electric field which are quite similar to the ones caused by our fish’s own body movement. This would mean that, when the electric fish is moving, it would be confused between signals caused by its own movement and the ones caused by the food, and therefore it won’t be able to feed at all.
Luckily for the electric fish, its brain came up with a mechanism able to cancel out the electrical perturbations caused in its body receptors by its own movement, while preserving the information that these receptors receive from prey. Own-body signal perturbations are typically perceived by all receptors in the fish’s body (thus they are usually called global signals), and they activate an additional group of neurons in the brain that generate an exact copy of this global signal, but inverted (peaks in the original signal will be troughs in the inverted signal, and vice versa). The original and inverted signals are then transmitted to a group of sensory neurons which add up these two signals. The signals, as a keen reader would already know, cancel each other out and this results in a “flat” response of the sensory neurons. This process does not occur for the prey-related signals, since they only stimulate the body receptors close to the prey, and therefore are not able to activate the “inverted signal” mechanism.
Interestingly, recent experimental and modeling studies have characterized how this mechanism holds for a wide variety of realistic conditions. For instance, the particular frequency of the modulations caused in the electric field by the stimulus can vary broadly. A study by researchers at the University of Ottawa in Canada demonstrated via in vivo recordings that the fish’s brain possesses a particular circuit for each frequency that needs to be canceled 2, so each frequency can be specifically suppressed by a given circuit. A more recent study 3, in which I personally contributed, tackles the problem of the signal having different intensities (which would correspond to the prey being located at different distances from the electric fish). It was found that the population of neurons responsible for generating the “inverse signals” modulate their activity accordingly to provide a properly scaled inverted signal which would match exactly the original one.
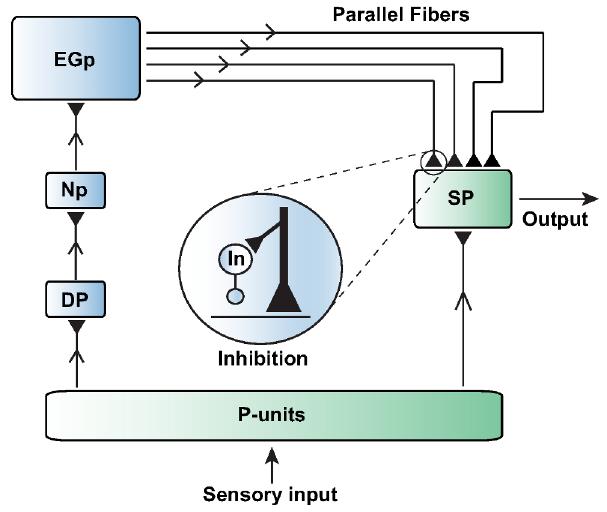
This strategy for canceling redundant information (body movement) in favor of behaviorally meaningful information (food) is fairly similar to the one used by the noise-cancellation headphones you use on airplanes: the headphones detect the environmental noise and generate an inverted signal, so that both of them cancel out and you can enjoy your music, the same way than the fish can enjoy its food. One could say that, somehow, natural competition found a way to build noise-cancellation headphones long before us…
References
- T. Requarth and N. B. Sawtell, Neural mechanisms for filtering self-generated sensory signals in cerebellum-like circuits. Curr. Op. Neurobiol. 21, 602-608, 2011. ↩
- K. Bol et al., Frequency-tuned cerebellar channels and burst-induced LTD lead to the cancellation of redundant sensory inputs. J. Neurosci. 31, 11028–11038, 2011. ↩
- Mejias J.F., Marsat G., Bol K., Maler L., Longtin A. & Gutkin B.S. (2013). Learning Contrast-Invariant Cancellation of Redundant Signals in Neural Systems, PLoS Computational Biology, 9 (9) e1003180. DOI: 10.1371/journal.pcbi.1003180.t002 ↩
1 comment
[…] En las profundidades marinas existen peces eléctricos, que usan las perturbaciones del campo eléctrico que generan para detectar a sus presas. Pero, podemos preguntarnos, ¿cómo hacen para no confundirse con las señales eléctricas que ellos mismos emiten al moverse? […]