How to engineer bacteria to treat cancer
It all began in 1891, when Dr. William B. Coley, a bone sarcoma surgeon at the Memorial Hospital in New York, injected streptococcal organisms into a patient with inoperable cancer. He thought that the infection he induced would have the side effect of shrinking the malignant tumor… and quite surprisingly he was right! The patient’s tumor was reduced, presumably because it was attacked by the immune system that was potentiated by the bacterial injection. This is the first rudimentary version of what we call today immunotherapy, a treatment that uses certain parts of a person’s immune system to fight diseases.
However, Coley’s therapies were highly criticized at that time because they were difficult to understand and had variable and unpredictable results. Also, radiotherapy was developed at the beginning of the 20th century, showing good results in fast tumor destruction and pain relief, and therefore Coley’s therapies were gradually displaced. Today, however, we can understand how Coley was able to see very good results: anaerobic bacteria (like the one he was using) tend to proliferate inside tumors due to their necessity to grow without oxygen. In addition, immune system cells such as macrophages and neutrophils cannot enter easily inside solid tumors and therefore bacteria can grow without the fear of being phagocyted 1. This last part is important; macrophages and neutrophils do a great job killing bacteria. You should not miss a very famous video in biology showing a neutrophil chasing a bacterium all over the dish until it reaches it and phagocytes it.
Video 1. Neutrophils are one of the first-responders of inflammatory cells to migrate towards the site of inflammation and are capable of ingesting microorganisms or particles. In the video we can see how the neutrophil (big cell) tries to reach the bacterium (small cell), which tries to run away.
However, the usage of regular bacteria to treat cancer is very limited since there is a clear trade-off between safe and effective dose (e.g., you would need a lot of bacteria to kill tumors but that can also kill yourself). Therefore, there is an important need to increase effectiveness and safety of these treatments. Luckily, nowadays researchers think this problem could be solved with synthetic biology.
Synthetic biology is the scientific field that designs and constructs biological devices, biological systems, and biological machines for useful purposes. In this sense, since bacterial genetics can be easily manipulated, synthetic biology techniques can be used to solve many of the key challenges that are associated with bacterial-mediated cancer therapies such as toxicity, stability and efficiency, and can be used to tune their beneficial features for cancer treatment 2. The idea would be that we could alter some bacterial genes to tune these bacteria intotiny programmable anticancer ‘robot factories’ that specifically target tumors (they don’t go everywhere in the body), selectively kill cancer cells (and not regular healthy cells), respond to external signals (to control bacteria inside bodies) and are externally detectable (to report where they are).
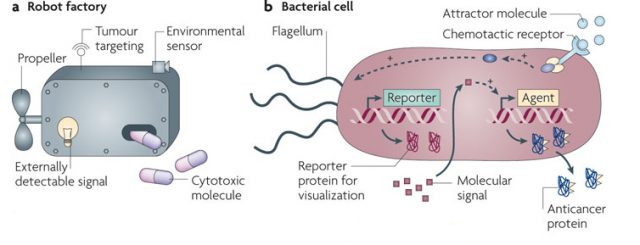
This looks nice as a theory, but there is increasing experimental data showing that the use of engineered bacteria is possible for treating cancer and other diseases. In the case of cancer, many different bacterial strategies have been implemented in animal models and some human trials have been carried out 3. Many studies have observed experimental success, with reduced tumor volume and increased survival and treatment of metastatic disease. One interesting approach was the use of bacteria that were forced to produce cytokines, molecules that are known to activate the immune system. Since bacteria accumulate and replicate within solid tumors, they produce these cytokines locally in the tumor environment potentiating the immune system to act in that particular region 4. With the same strategy, bacteria have been engineered to produce toxins, which locally would kill tumor cells but no other cells in the body 5. Another smart example has been the use of bacteria as pro-drug converters. Pro-drugs are precursors of drugs that are not effective until they are modified in some way. Therefore, bacteria have been engineered to be able to convert pro-drugs in effective drugs, helping to treat only the tumor and reducing side effects of the drug in the rest of the body, one of the main caveats of chemotherapy these days 6.
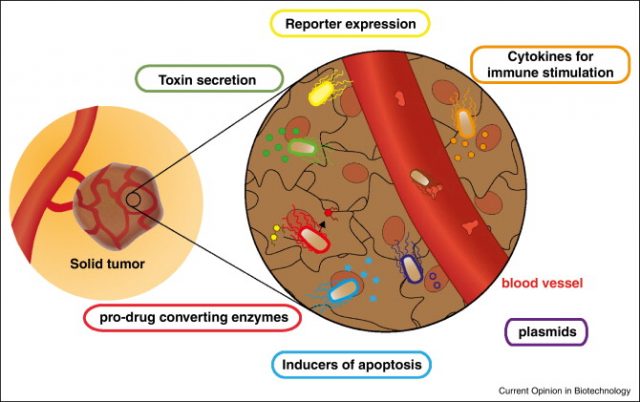
However, all of these approaches depend on the correct localization of the bacteria into the tumor. To improve localization, a group from the Spanish National Biotechnology Center modified bacterial adhesins to target tumor surfaces. Adhesins are bacterial cell-surface components that facilitate adherence to other cells or to surfaces. Therefore, researchers created new synthetic adhesins that detect and adhere to tumoral cells specifically, increasing rate localization and reducing the amount of bacteria needed for tumor colonization7.
All of these experiments and many more have shown the potential for bacteria to treat cancer and other diseases. Given the flexibility of bacterial genetics, the ultimate bacterial therapy will consist of a collection of strains designed for specialized purposes that could act cooperatively and in combination with molecular chemotherapy. Once perfected, anticancer bacteria are predicted to be an essential clinical tool that will be able to carry out functions unachievable by other therapies, helping to treat tumors and metastases in a new different way.
References
- Westphal, K., Leschner, S., Jablonska, J., Loessner, H., & Weiss, S. (2008). Containment of tumor-colonizing bacteria by host neutrophils. Cancer research,68(8), 2952-2960. doi: 10.1158/0008-5472 ↩
- Forbes, N. S. (2010). Engineering the perfect (bacterial) cancer therapy. Nature Reviews Cancer, 10(11), 785-794. doi:10.1038/nrc2934 ↩
- Piñero-Lambea, C., Ruano-Gallego, D., & Fernández, L. Á. (2015). Engineered bacteria as therapeutic agents. Current opinion in biotechnology, 35, 94-102. doi:10.1016/j.copbio.2015.05.004 ↩
- Loeffler, M., Le’Negrate, G., Krajewska, M., & Reed, J. C. (2007). Attenuated Salmonella engineered to produce human cytokine LIGHT inhibit tumor growth. Proceedings of the National Academy of Sciences, 104(31), 12879-12883. doi: 10.1073/pnas.0701959104 ↩
- Nguyen, V. H., Kim, H. S., Ha, J. M., Hong, Y., Choy, H. E., & Min, J. J. (2010). Genetically engineered Salmonella typhimurium as an imageable therapeutic probe for cancer. Cancer research, 70(1), 18-23. doi: 10.1158/0008-5472 ↩
- Lehouritis, P., Springer, C., & Tangney, M. (2013). Bacterial-directed enzyme prodrug therapy. Journal of Controlled Release, 170(1), 120-131. doi:10.1016/j.jconrel.2013.05.005 ↩
- Piñero-Lambea, C., Bodelón, G., Fernández-Periáñez, R., Cuesta, A. M., Álvarez-Vallina, L., & Fernández, L. A. (2014). Programming Controlled Adhesion of E. coli to Target Surfaces, Cells, and Tumors with Synthetic Adhesins. ACS synthetic biology, 4(4), 463-473. doi:10.1021/sb500252a ↩
1 comment
[…] Sourced through Scoop.it from: mappingignorance.org […]