Mimicking animals to improve device properties
Author: Daniel González-Muñoz is a predoctoral researcher in photocatalytic processes at Universidad Autónoma de Madrid.
If you are a fan of the MARVEL universe, you will have noticed that many of its characters, such as Spiderman, Black Panther, Ant-Man or Wolverine, have superpowers based on the abilities of certain animals. Using the animal kingdom as a reference, and using the abilities of certain animals, is not something exclusive to comics. Science has been observing nature for thousands of years, understanding the processes that govern it and trying to imitate it. This helps us improve the technology of our society, connecting with the environment that surrounds us.
Well, if I now tell you that the properties of the animals that we are going to imitate are mussels and chameleons, you might be a little disappointed. Do not feel this way! These properties are more interesting than what you might imagine. Specifically, this study tries to imitate the ability chameleons have to change colour with the medium and the adhesion capacity of mussels. These properties are of great interest in flexible electronic devices for health monitoring or sensory skins 1
To mimic the adhesion capacity, they use a hydrogel that is gaining a lot of attention due to its structural similarity to the adhesive proteins secreted by mussels: polydopamine (PDA). Chameleons are able to change the colour of their skin thanks to a network of guanine nanocrystals that respond to photon changes. To mimic this characteristic, a flexible hydrogel, called polyurethane (PU), with an inverse opal-structure is used. With these two polymers it is possible to make a flexible device, which is capable of changing colour and is also adhesive and self-healing. The authors of this study go further and add the factor of electrical conductivity by inserting carbon nanotubes (CNTs), which are capable of changing the resistance of the device when stretched (Figure 1).
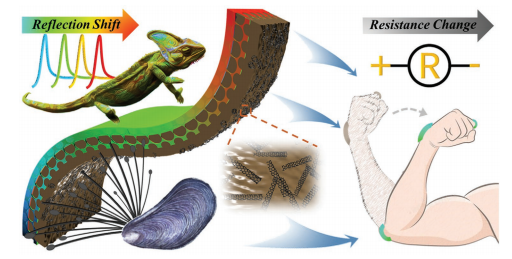
To build this device, a hexagonal network of silica nanoparticles is first created (Figure 2a(i) and 2b). Then, a PU solution is added that covers the holes in the hexagonal structure. After removing the silica network, the inverse opal-structure remains (Figure 2a(ii) and 2c-d). Finally, a mixture of PDA-CNTs interpenetrated with polyacrylamide (PAAm) is introduced. The PDA-CNTs act as the conductive and adhesive layer while the PAAm acts as the base layer of the device (Figure 2a(iii) and 2e-g).
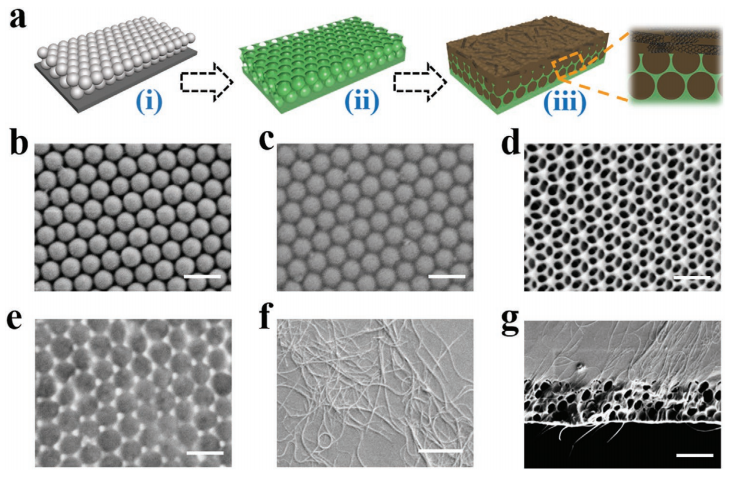
Due to the structure of the PU film, a unique optical property is given as a result of the photonic band gap (PBG). This phenomenon is responsible for the bright colour of the device and means that radiation of a given wavelength cannot propagate and is reflected. This reflected wavelength is given by Bragg’s law (Eq 1), where λ is the reflection peak wavelength, d is the center-to-center distance between nanoparticles, and naverage is the average refractive index of the material. This means that larger silica nanoparticles – used as template – lead to larger the holes in the PU film structure, greater values for d, and therefore, a larger reflected wavelength λ.
λ=1.633dnaverage (Eq 1)
Another important factor to take into account is the amount of CNTs added. These nanomaterials are conductors, and larger amounts decrease the resistance of the device. However, a high amount of CNTs reduce the stretchability of the structure, so the optimal amount of CNTs was studied and set at 50% vol.
Once all the synthetic parameters were optimized, the different properties of the device were measured. As you can see in figure 3, different stretching experiments were carried out. Firstly, as stretchability increases, the colour of the device changes (Figure 3a-c). As we saw in Eq 1, modification of the PU structure changes the parameter d. When stretching the device, the structure is compressed, and therefore, d decreases. This implies that the reflection wavelength also decreases (blue shift), causing the device to present an increasingly blue colour (Figure 3d). In addition, another stretch-dependent parameter, current resistance, is measured. As you can see in figure 3e, stretching the device leads to greater resistance to current. With these two experiments, they show that the device features two different responses to physical stimulus (stretching), by changing colour and becoming more resistant to electric current.
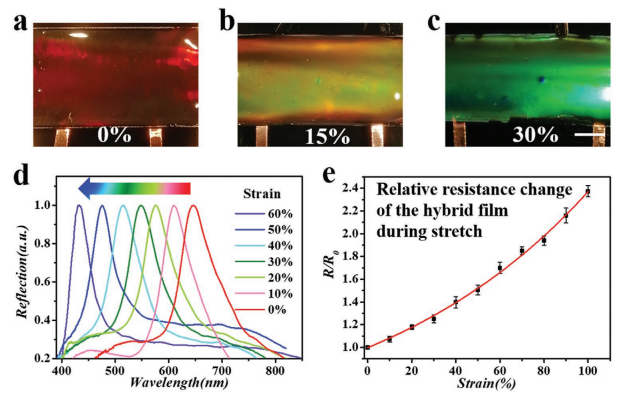
As you can see, the characteristics of these devices are amazing, but there is still more. Taking advantage of the magnificent properties of PDA, the device was endowed with adherent and self-healing properties. To demonstrate its adherent properties, the device was attached to porcine skin (Figure 4a). The device demonstrated great adhesion even when the porcine skin was folded at different angles. When the device was detached from the skin, hardly any residue was observed, demonstrating its great ability as an adherent device. The self-healing ability can be seen in figure 4b. By joining two different devices, in just two hours they present a complete union between them. And the effectiveness of this union was verified by subjecting the device to strains (Figure 4c), showing that the device could withstand the stretch without breaking. And this self-healing ability goes further. Not only is it demonstrated by joining two rectangular devices, which is a very simple joint, but different devices can be joined to generate much more complex shapes. In figure 4d you can see how four shapes with very different edges can be joined together to form a four-leaf clover completely resistant to strains.
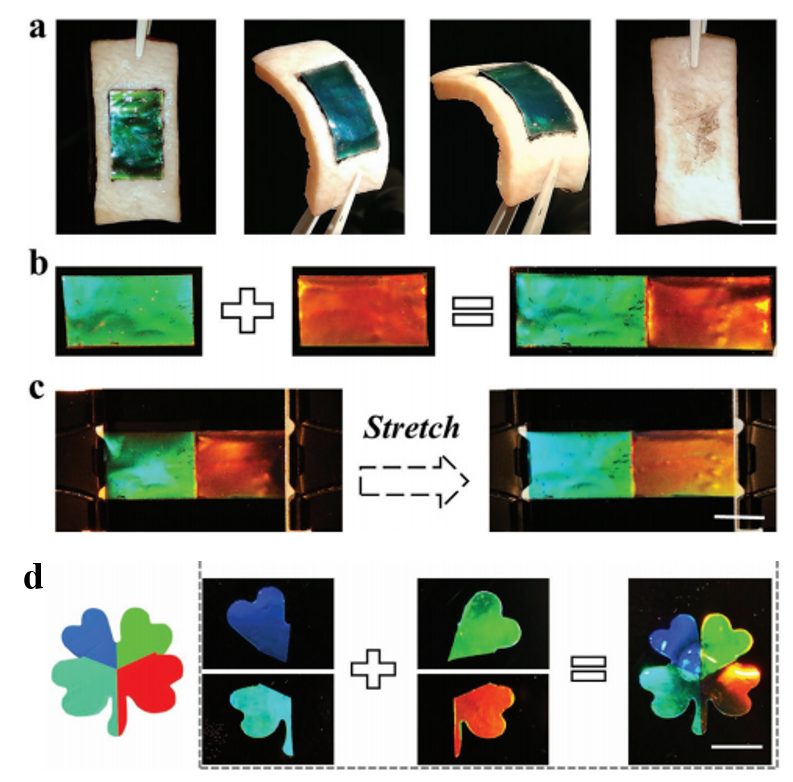
These devices have been shown to have adhesion properties, self-healing ability, conductivity, and colour-based sensing ability. To finish this study, they showed how these devices can be used with flexible devices in human joints. Specifically, the device was tested on a finger (Figure 5a), a wrist (Figure 5b) and an elbow (Figure 5c). The change in the reflection wavelength was measured at different angles (Figure 5d-f), observing that the greater the angle at which the joint was bent led to a greater displacement in the reflection wavelength. In addition, the change in resistance was also measured (Figure 5g-i), observing the same pattern: the greater the angle at which the joint was bent, the greater the current resistance of the device.
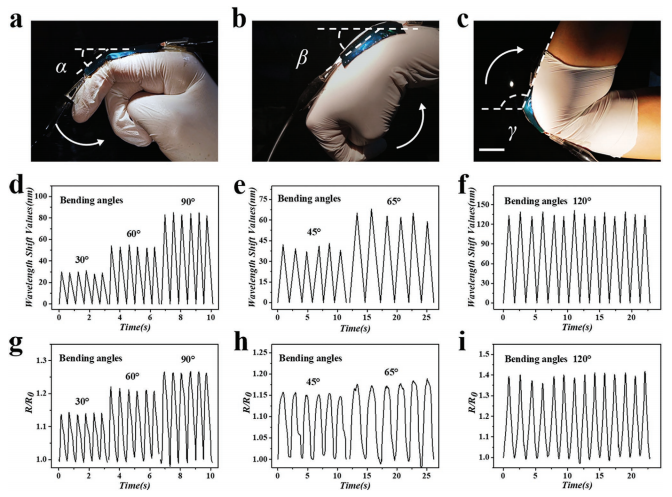
The results of this study have showed us a flexible electronic device capable of adhering to different joints of the human body, with self-healing capacity and which is capable of responding to the stimuli of strains by changing colour and modifying the resistance to electric current. This shows that nature holds incredible secrets. If we are able to observe it, learn from it and imitate it, the future that we can achieve with technological advances will have no limit.
References
- Yu Wang, Yunru Yu, Jiahui Guo, Zhuohao Zhang, Xiaoxuan Zhang and Yuanjin Zhao (2020) Bio-Inspired Stretchable, Adhesive, and Conductive Structural Color Film for Visually Flexible Electronics. Adv. Funct. Mater. 2000151. DOI: 10.1002/adfm.202000151. ↩
1 comment
[…] Elektronikan gauzarik bitxienak egiteko inspirazio izan daitezke animaliak. Kolorea aldatzeko gaitasuna (kamaleoian oinarrituta) eta itsastekoa (muskuiluan), adibidez. Daniel González Muñozen eskutik: Mimicking animals to improve device properties […]