Converting photons from visible to UV to treat water
Author: Daniel González-Muñoz is a predoctoral researcher in photocatalytic processes at Universidad Autónoma de Madrid.
You are surely familiar with the concept that light behaves like electromagnetic radiation, and that it responds to the equation In this way, the higher the wavelength of the light, the lower its frequency, and vice versa. This means that if we compare red and blue light, for example, we will see that red light has a longer wavelength than blue. However, blue light has a higher frequency than red light (Figure 1). Furthermore, this frequency is associated to an energy by the equation. This means that the photons of light do not only have a wavelength and a frequency associated with them, but also an energy (E). Understanding these concepts is key to being able to analyse electromagnetic radiation from the Sun. This electromagnetic radiation is composed of ultraviolet (UV, 5%), visible (43%) and infrared (52%) radiation, so applications that are developed using solar radiation will be dependent on the range of radiation that can be absorbed.
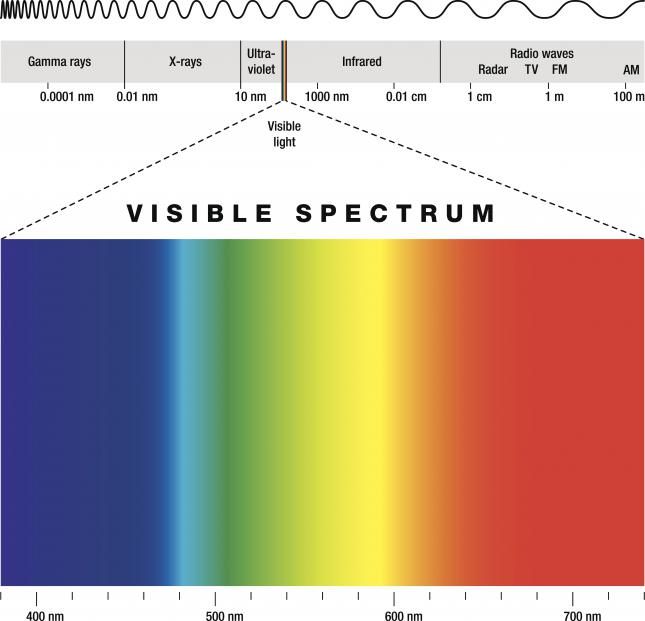
One of the most promising applications within solar applications is water decontamination by photocatalysis, which uses molecules capable of absorbing solar radiation (photocatalysts) and transmitting it to other molecules to trigger their degradation. In the context of water decontamination, pollutants present in wastewater, such as viruses, bacteria or common drugs (paracetamol) can be readily degraded. While this goal seems straightforward, its execution carries some problems. On the one hand, to degrade some of these pollutants, highly energetic radiation (UV) is needed, which is the least abundant in the solar spectrum (5%). On the other hand, using photocatalysts capable of absorbing visible light (43% of the solar spectrum) would allow for a better use of the solar spectrum, but the radiation is not sufficiently energetic.
In light of these issues, could there be a solution to solve both problems? Insert one of the most amazing mechanisms in photocatalysis: the triplet-triplet annihilation (TTA) (Figure 2, top). In this phenomenon there are two main protagonists: sensitizers (S), molecules capable of absorbing visible light, and annihilators (A), capable of capturing the energy that sensitizers have previously absorbed. The first step is the absorption of visible light by two sensitizer molecules. This absorbed energy causes the electrons in these molecules to promote to an excited level (1S). Then, the electrons transition to a lower-energy triplet state (3S). At this point, a triplet-triplet energy transfer (TTET) occurs between the two sensitizer molecules and two annihilator molecules. The energy absorbed by the sensitizers is transferred to the annihilators, which promotes their electrons to a triplet excited state (3A), while the sensitizers, having released the energy, return to their ground state (S). The two annihilators in the 3A triplet state give the triplet-triplet annihilation, which consists of using the energy absorbed by the two annihilators to promote an electron to a highly energetic single excited state (1A) in one annihilator, while the other annihilator returns to its ground state (A). Finally, this excited state 1A is capable of acting as a reductant, and in this case, reducing and degrading certain pollutants. This process may be a bit complicated to assimilate, but the main idea is that by using visible light (2.8 eV) we can reach excited states of higher energy (3.9 eV) due to the TTA up-conversion, while this highly energetic state could only be accessed through direct catalyst excitation with UV radiation. In this way we can use visible radiation, which is more abundant in the solar spectrum, and convert its photons into radiation as energetic as UV.
In this work 1, four different iridium photocatalysts – acting as sensitizers – and two annihilators have been synthesized (Figure 2, bottom). Importantly, both sensitizers and annihilators have anionic substituents in their structure. This is necessary for the molecules to be soluble in aqueous medium.
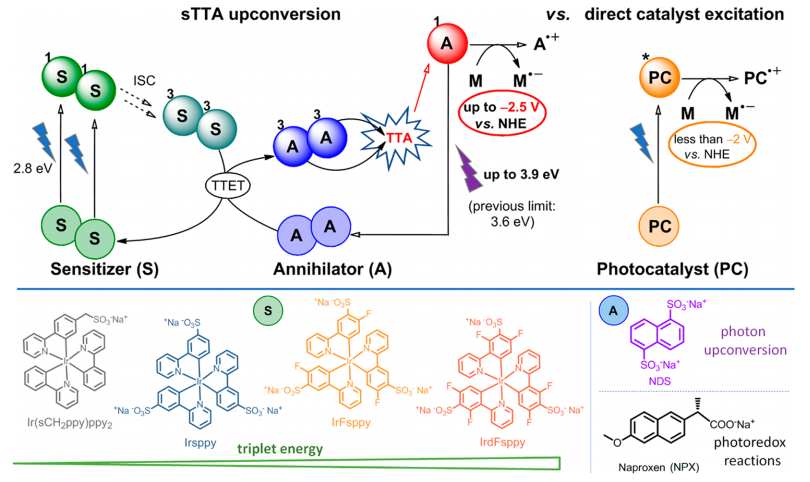
The authors studied the photophysical properties of the iridium sensitizers. The absorption and emission spectra for the four sensitizers are represented in Figure 3. By inserting fluorine atoms in their aromatic rings, an increase in the energy of the triplet state is observed (2.50 to 2.81 eV). This is clearly seen in the emission spectra, since the emission peak undergoes a blue shift. Furthermore, the lifetime of this excited state also increases, but the extinction coefficient, which is the amount of light absorbed per concentration unit, decreases. Although all four iridium compounds have good sensitizing properties, Irsppy was chosen for the pollutant degradation experiments since Ir(sCH2ppy)ppy2 presents the lowest triplet state energy to carry out TTET, and IrsFppy, IrdFsppy exhibited less stability.
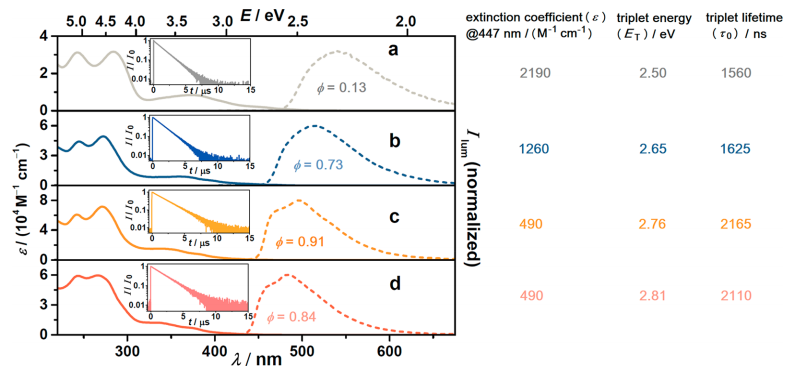
As for the annihilators, they calculated their energy from the triplet state (3A) at 2.58 eV. However, the energies of their excited singlet states (1A) were calculated at 3.9 eV for NDS and 3.7 eV for NPX. Knowing this data and the ground state oxidation potentials, the excited state reduction potentials (E1/2(A·+/1A*)) could be calculated. As shown in Figure 2, the NDS annihilator has two electron withdrawing groups, while NPX has one electron donating group. This makes the electrons more concentrated in the aromatic structure of NPX and thus easier to oxidize. Therefore, its excited state reduction potential is going to be more negative than NDS (Equations 1, 2). For this reason, NPX was selected to carry out the degradation experiments of contaminants in water.
Equation 1: E1/2 NDS(A·+/1A*) = -1.9V
Equation 2: E1/2 NPX(A·+/1A*) = -2.5V
To test the effectiveness of TTA on a laboratory scale, the Irsppy sensitizer and the NPX annihilator were used in the reduction of 4-bromo-2-chloro-5-fluoro-benzoate in aqueous solution under visible light in just 2 hours (Figure 4). It is important to note that the reduction of the bromide functionality in this molecule is especially difficult due to its high reduction potential. However, thanks to the TTA mechanism, a yield of 63% of the debrominated product was obtained. Furthermore, isopropanol is added to the solution since it can act as a hydrogen donor once the aryl radical is formed to obtain the final product.
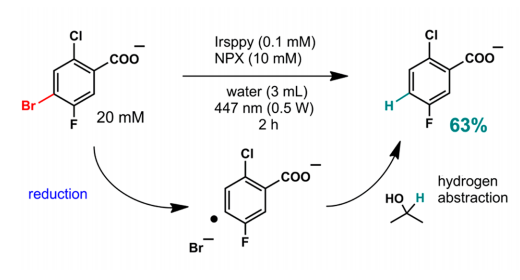
Finally, the degradation of a real pollutant was studied. The benzyltrimethylammonium cation is a common basic structure which represents the class of quaternary ammonium substance compounds (QAC) (Figure 5). These compounds are potentially dangerous for the environment and require highly energetic radiation to be degraded. Thanks to TTA this degradation can occur using visible light. As shown in Figure 5, irradiating NPX with energetic light (<340 nm) can degrade the compound. However, using Irsppy as a sensitizer, TTA can be carried out and completely degrade the contaminant under visible light (455 nm) in 23 hours.
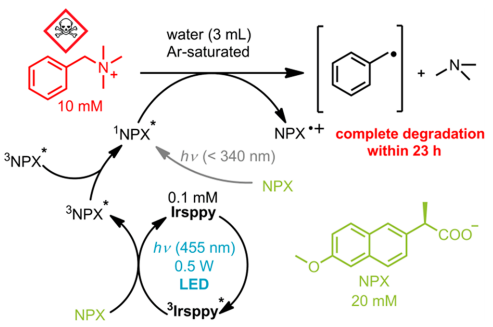
This study shows us the potential of photocatalysis as a tool to decrease environmental pollution while using solar radiation as the lone energetic source. It also shows that it can be applied in real-life situations and that photocatalysis can be considered as one of the most promising and sustainable processes to be used in the coming years.
References
- Björn Pfund, Debora M. Steffen, Mirjam R. Schreier, Maria-Sophie Bertrams, Chen Ye, Karl Börjesson, Oliver S. Wenger and Christoph Kerzig (2020) UV Light Generation and Challenging Photoreactions Enabled by Upconversion in Water. J. Am. Chem. Soc. 142, 10468-10476. DOI: 10.1021/jacs.0c02835 ↩
3 comments
I just want to say: Thank you for highlighting our work.
We are still working on related systems, so stay tuned.
All the best,
Christoph
Hi Christoph,
Thank you very much for your words. In my opinion, it is a very original work with a lot of potential for the future.
Best regards.
[…] Erradiazio ultramorearekin kontsumorako ura tratatu badaiteke, baina erradiazio mota hau ez badago beti erabilgarri, zergatik ez bihurtu erradiazio ikusgarria ultramore? Prozesu innobatzaile eta burutsua. Daniel González-Muñozen Converting photons from visible to UV to treat water […]