A different class of van der Waals magnets
The so-called van der Waals materials consist of two-dimensional layers bound by weak van der Waals forces. After the isolation of graphene, the field of two-dimensional van der Waals materials has experienced an explosive growth and new families of two-dimensional systems and block-layered bulk materials, such as tetradymite-like topological insulators – electronic materials that have a bulk band gap like an ordinary insulator but have conducting states on their edge or surface.
This growth has been fuelled mainly by the possibility of tuning a set of remarkable electronic properties using an array of methods (thickness control, doping, intercalation, proximity effects, to name a few) coupled with a relative simplicity of fabrication. These two characteristics are what make the layered van der Waals materials attractive from both practical and fundamental points of view.
However, the important step towards magnetic functionalization of the inherently nonmagnetic layered van der Waals materials and a controllable fabrication of the resulting hybrid systems has proven challenging. As a matter of fact, the field of two-dimensional van der Waals magnets is in its infancy and many more materials with new properties are to be explored. In particular, van der Waals antiferromagnets are expected to be of great interest. Antiferromagnetism occurs below a certain temperature, named after Louis Néel, when an ordered array of atomic magnetic moments spontaneously forms in which alternate moments have opposite directions. There is therefore no net resultant magnetic moment in the absence of an applied field.
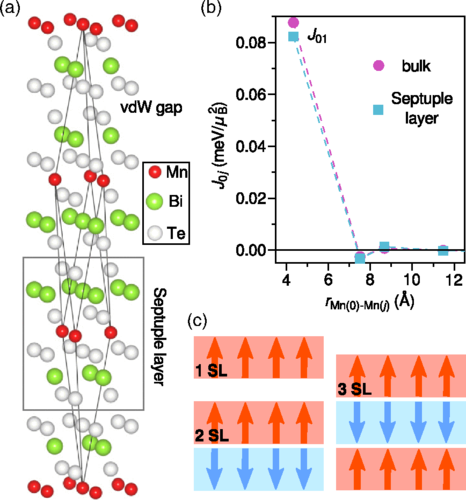
The layered van der Waals compound MnBi2Te4 was reported a few years ago as the first ever antiferromagnetic topological insulator. MnBi2Te4 (MBT) and related compounds MnBi2nTe3n+1 are synthesized materials that combine electronic topology with intrinsic magnetic order (i.e., not via
dilute magnetic dopants). Now, a team of researchers has investigated 1 magnons (also called spin waves, magnons are collective oscillations associated with magnetic systems) and magnetic fluctuations in MBT in the ultrathin thickness limit using Raman spectroscopy measurements.
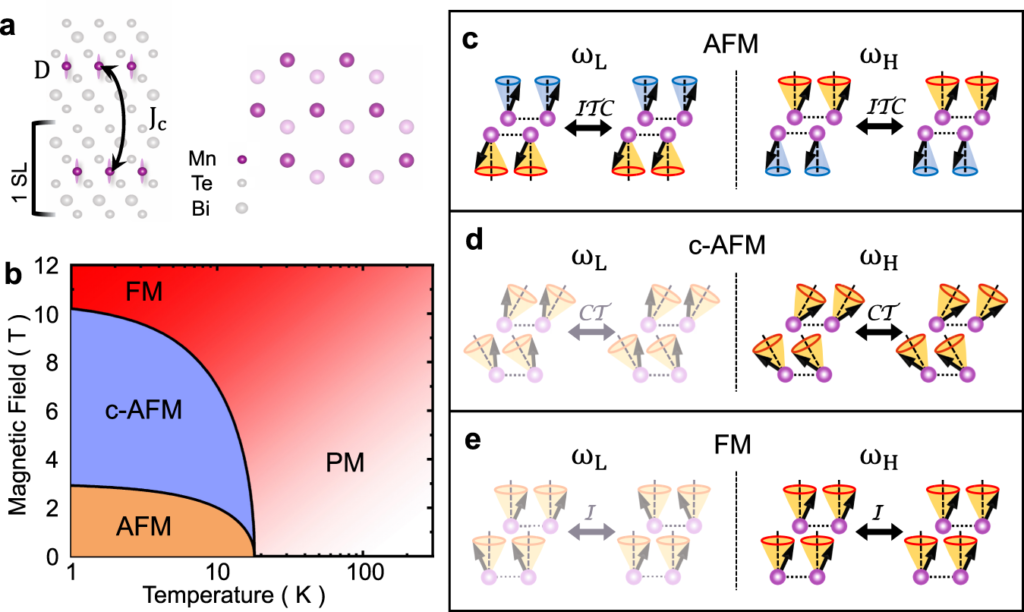
While the electronic topological properties originate from the Bi and Te layers, the long-range spin ordering is attributed to the magnetic Mn2+ ion layers. The interplay between magnetism and electronic topology has been shown to be thickness-dependent. Although single septuple layer MBT is topologically trivial, two or more septuple layers present distinct topological phases according to their magnetic order and number of layers. At low temperature, the Mn moments in each septuple layer are ferromagnetically ordered while the Mn moments in the adjacent septuple layer exhibit an antiferromagnetic order, leading to a vanishing net magnetic moment in samples with an even number of septuple layers.
The researchers focused on two septuple layers partially because it represents the thinnest sample in which MBT exhibits all three possible magnetic orders: antiferromagnetic, canted-antiferromagnetic, and ferromagnetic when an external magnetic field perpendicular to the 2D plane is tuned. They observed a long-wavelength magnon mode at low temperature under both zero and finite external magnetic fields and in all three magnetic phases, revealing the formation of long-range magnetic order. In the antiferromagnetic phase, the unusual selection rule and the lack of magnetic field-dependent frequency shift suggest that two-magnon scattering leads to the observed mode.
Using spin-wave theory, the authors calculate the spin-wave gap at zero magnetic field with values for anisotropy energy and interlayer exchange in the bilayer. The energetic terms as well as the magnon selection rules suggest that MBTs are in a different class of van der Waals magnets from the widely studied CrI3. Magnetic fluctuations in the few-layer samples and a bulk crystal were found to increase with decreasing layer thickness, which may contribute to a less robust magnetic order in single layers.
Author: César Tomé López is a science writer and the editor of Mapping Ignorance
Disclaimer: Parts of this article may have been copied verbatim or almost verbatim from the referenced research paper/s.
References
- David Lujan, Jeongheon Choe, Martin Rodriguez-Vega, Zhipeng Ye, Aritz Leonardo, T. Nathan Nunley, Liang-Juan Chang, Shang-Fan Lee, Jiaqiang Yan, Gregory A. Fiete, Rui He & Xiaoqin Li (2022) Magnons and magnetic fluctuations in atomically thin MnBi2Te4Nature Communications doi: 10.1038/s41467-022-29996-w ↩