New insights on methane’s effects on climate warming
methane
Author: Rubén Soussé Villa is pursuing a PhD in atmospheric chemistry at Barcelona Supercompting Center / Universitat Politècnica de Catalunya
Methane (CH4) is the most powerful greenhouse gas (GHG) after CO2. It is widely used but also feared, because a single tonne of CH4 emitted today can heat up the atmosphere up to 30 times what a tonne of CO2 in the next 100 years 1. Because of its huge heating power but its relatively short permanency in the atmosphere (about a decade), reducing its emissions is presented as a hopeful fast way to tackle climate change 2.
However, a recent study 3 quantifying the warming effect of methane has found slightly lower values than those calculated until now. The study considered additional methane’s climate effects beyond its direct interaction with radiation, including Earth’s adjustments to these interactions, and found that methane could have up to 30% less heating capacity as previously thought. Although it does not mean that methane is not still a very powerful methane gas, this study could provide an additional insight on how methane greenhouse effect is calculated in climate models.
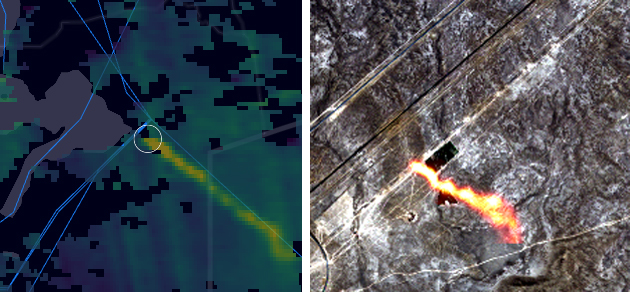
Methane is an odorless and colorless gas emitted from many different anthropogenic and natural sources, such as the energy industry, livestock (from digestion of organic matter), rice crops plus other flooded fields and landfills (through anaerobic decomposition of organic matter), etc.. All these sectors have grown massively since pre-industrial times (1850), and consequently methane concentration in the atmosphere have more than doubled since then, rising from 720–740 parts per billion by volume (ppb) to 1800 ppb, so a factor of 2.4 45. It is estimated that methane alone heated up climate by 0.24 K, almost a quarter of the 1 ºC temperature increase since pre-industrial times.
Methane heats climate through, principally, its strong absorption of the long wavelength radiation (LWr) emitted from the Earth’s surface in the infrared (IR). Absorbing LWr, methane prevents this radiation leaving Earth’s atmosphere, trapping heat into it and warming up the climate, as all GHGs. Additionally, methane absorbs Sun’s short-wavelength radiation (SWr) in the near-infrared band (nIR). The absorption of SW has been found to also warm the atmosphere. SWr absorption increases the warming effect of methane by 7 to 15% compared to the LWr absorption alone. These two effects, the absorption of LWr plus SWr, can be called the direct climate effect of methane; They represent methane’s direct interaction with radiation. It is the most studied until now and it is estimated to, overall, heat up the Earth. However, the climate reacts to this heating in different ways, called adjustments (ADJs). These ADJs can warm or cool the climate, adding to or counterbalancing radiation warming. Therefore, only taking into account methane’s direct climate effects (LWr and SWr absorption), methane is a very powerful GHG. But this might be an incomplete picture, since climate ADJs might reduce this power. And specifically, climate ADJs to SWr absorption are not always incorporated in climate models.
How can methane’s climate ADJs be quantified? To start with, it is needed a climate model that simulates an atmosphere with methane alone (no other GHGs, to isolate the sole effect of methane). It should include also ocean dynamics (internal circulation, temperature, etc.), cloud dynamics and methane’s LWr absorption. This is the standard in most climate models. Additionally, it needs to count for methane’s SWr in the nIR, which is not a common case in all models. The cited study used the Community Earth System Model version 2 (CESM2) for this purpose. With such a climate model, it is time for experimenting. Several identical simulations are performed, except that some include methane’s LWr absorption alone and some LWr and SWr absorption. For each case, three additional simulations are done, one with the complete simulation of ocean dynamics, one with fixed sea-surface temperature but no ocean internal circulation, and one with no ocean considered. These three extra simulations are important, because they are useful to assess separately which climate factors are more impacted by methane’s radiation. Finally, to assess the effects of methane’s emission increases, each simulation is run with 2, 5 and 10 times the methane’s atmospheric concentration in pre-industrial times (i.e. 750ppm). Once all simulations are performed, the response of climate to each methane concentration is analyzed from the information derived from all of them. Since they are all different, they provide different information regarding different climate factors (for example clouds, surface temperature, air temperature, etc.).
To begin with, from simulations reproducing complete ocean dynamics, it is obtained that climate ADJs to methane’s SWr absorption offset its climate warming from LWr absorption by 30%. Present-day methane concentration (x2.4 times pre-industrial levels) climate effects are inferred from the different methane’s concentration simulations (x2, x5 and x10 times). In this case, the study found that the “default” LWr warming of methane – estimated at 0.20 ºC – would be lowered by 0.04 ºC if SWr absorption is considered. Also, precipitation is affected if SWr absorption is included in the model, decreasing the precipitation rate in 60%.
Why does it happen? The study’s different simulations allow a sequential break-down of methane’s climate effects. Apparently, it indicates that, regarding only radiation, SWr absorption by methane still contributes to heat the climate. However, the ADJs in the atmosphere as a consequence of SWr absorption are what drive an offsetting of the purely radiative heating due to LWr and SWr absorption. Specifically, it is a vertical redistribution of cloud formation in the atmosphere what halts climate warming (figure 2).
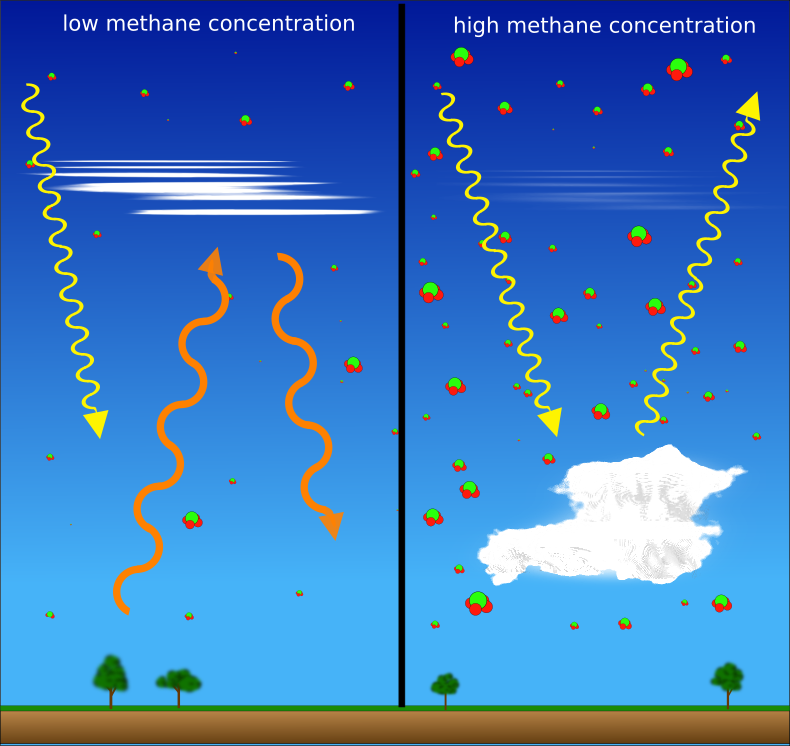
How are clouds affected by methane? Methane’s SWr absorption happens in three bands in the spectrum that are actually shared with water vapor, which is another GHG by the way. Water vapor is very abundant in the lower troposphere (so near the surface) and scarce in the upper troposphere. Methane, on the other hand, is vertically equally-spread in the atmosphere. So it absorbs SWr in the upper troposphere, where water vapor concentration is low. Methane absorbing SWr in the high atmosphere causes two effects: 1) these high altitude layers heats up, this causes the decrease in the formation of high altitude clouds, which tend to heat the climate by preventing Earth’s LWr from leaving the atmosphere. Additionally, 2) methane absorbs the SWr before it reaches the surface, where water vapor would absorb it otherwise. If water vapor does not absorb SWr near the surface, then the temperature of this layer starts to drop. A lower temperature near the surface causes the opposite effect: clouds start to form. These low-level clouds tend to be thick, and are specially good at reflecting incoming solar light back to space. So the enhanced formation of these surface clouds tend to cool the climate. From the simulations’ results with fixed sea-surface temperature and with coupled ocean, it seems clear that clouds play a central role in decreasing the heating power of methane, clearly offsetting Earth’s tropospheric and surface temperature rise.
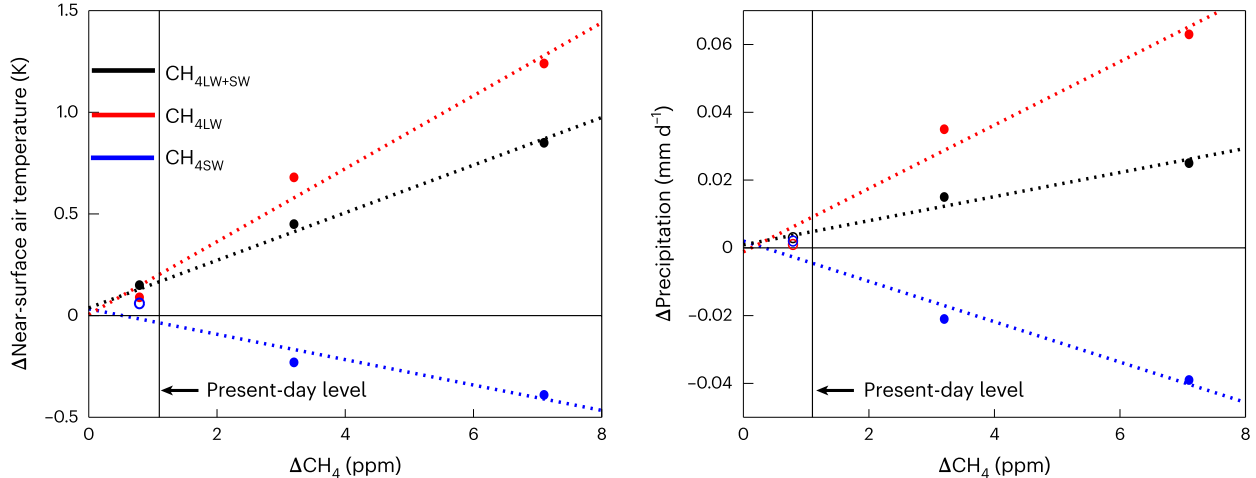
Regarding methane’s concentration effects, the study’s results are more relevant if assuming 10 times the pre-industrial methane concentration. Assuming double concentration – which is the current methane level – the effects are less visible (figure 3), but results with 10 times methane concentration still help us to approximately guess what to expect in a climate where methane concentrations are expected only to increase, and that might reach 5 times the pre-industrial level by the end of the century 6. And the results still represent that current 30% of climate warming and 60% of precipitation increase might be offset by methane’s secondary climate effects.
It is important to note that the study does not include methane’s indirect climate effects, such its contribution to enhance ozone formation, another powerful GHG. And after all, the results from the study do mean in any way that methane is a cooling gas. It only means that methane’s already very high heating capacity might be slightly lower than previously thought. But it is to be checked with other models and studies yet. And it does not mean that methane isn’t anymore the second most powerful GHG and that its emissions should be cut off urgently in order to avoid disastrous climate consequences.
More on the subject:
How to get 99% less methane emissions from the slurry pit
Geoengineering versus Global Warming
References
- IPCC. Climate Change 2018: The Physical Science Basis; Cambridge University Press: Cambridge, UK, 2018. ↩
- Szopa, S., V. Naik, B. Adhikary, P. Artaxo, T. Berntsen, W.D. Collins, S. Fuzzi, L. Gallardo, A. Kiendler-Scharr, Z. Klimont, H. Liao, N. Unger, and P. Zanis, 2021: Short-Lived Climate Forcers. In Climate Change 2021: The Physical Science Basis. Contribution of Working Group I to the Sixth Assessment Report of the Intergovernmental Panel on Climate Change [Masson-Delmotte, V., P. Zhai, A. Pirani, S.L. Connors, C. Péan, S. Berger, N. Caud, Y. Chen, L. Goldfarb, M.I. Gomis, M. Huang, K. Leitzell, E. Lonnoy, J.B.R. Matthews, T.K. Maycock, T. Waterfield, O. Yelekçi, R. Yu, and B. Zhou (eds.)]. Cambridge University Press, Cambridge, United Kingdom and New York, NY, USA, pp. 817–922, https://doi.org/10.1017/9781009157896.008. ↩
- Allen, R.J., Zhao, X., Randles, C.A. et al. Surface warming and wetting due to methane’s long-wave radiative effects muted by short-wave absorption. Nat. Geosci. 2023, 16, 314–320. doi: 10.1038/s41561-023-01144-z. ↩
- Etheridge, D.M.; Pearman, G.I.; Fraser, P.J. Changes in tropospheric methane between 1841 and 1978 from a high accumulation-rate Antarctic ice core. Tellus B 1992, 44, 282–294. ↩
- Derwent, R.G. Global Warming Potential (GWP) for Methane: Monte Carlo Analysis of the Uncertainties in Global Tropospheric Model Predictions. Atmosphere 2020, 11, 486. doi: 10.3390/atmos11050486 ↩
- Allen, R. J. et al. Significant climate benefits from near-term climate forcer mitigation in spite of aerosol reductions. Environ. Res. Lett. 2021, 16, 034010. ↩