Fit-to-purpose power sources: portable batteries ecodesigned within the planetary boundaries
Digital technologies have gained an essential role in societies’ development, by generating a global transformation in economic, social, and environmental dimensions. Digitalization does not just bring comfort and effectiveness in daily life but also contributes to equity and opportunities generation. However, this digital transformation is raising a fierce debate on how to ensure a positive, sustainable and just social impact.1–3
As society’s digital dependence speeds up, most products’ life cycles become shorter. The rapid technology evolution, together with the consumers’ demand, planned obsolescence and linear economy models, are leading to a rapid discard of the ‘old’ units.4 Such global production and consumption patterns are generating an immense quantity of waste of electrical and electronic equipment (namely, e-waste or WEEE). These discarded devices are the world’s fastest-growing solid-waste stream.5 According to the last report from the United Nations’ Global Transboundary E-waste Flows Monitor, in 2019, the global generation of electronic waste amounted to 53.6 million metric tons, averaging 7.3 kilograms per capita. Europe in particular ranks first in terms of e-waste generation per capita, with 16.2 kg per capita. Global predictions warn that by 2030, e-waste generation is projected to surge to 74.7 million metric tons and could potentially escalate to 110 million metric tons by 2050.4,6 On the other hand, the World Economic Forum estimates that the material value of our used electronic devices globally amounts to $62.5 billion.7 Thus, the e-waste stream equally contains hazardous and valuable materials. However, despite the efforts made by countries and organizations towards e-waste management, in 2019, the documented global percentage of collected and properly recycled WEEE was only 17.4%.4,8 When collected, these discarded devices are sent for recycling, where high-value components are recovered (whereas the low-value materials are simply disposed of in landfills).9 Still, the complex design and material heterogeneity make this recycling process really time-consuming and energy-intensive.10
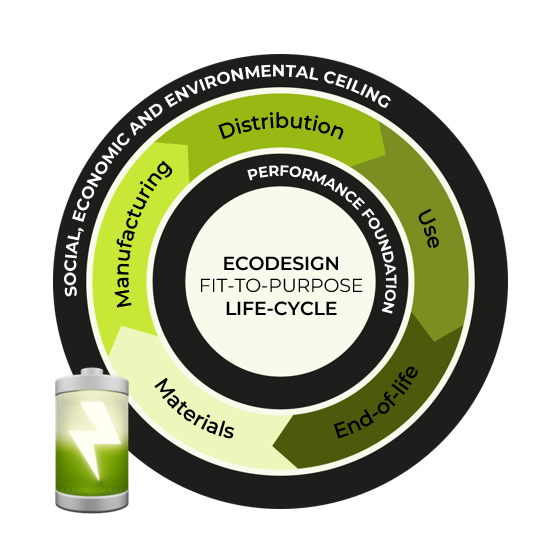
In this scenario, portable batteries play an essential role. Due to their capability to deliver high energy densities in a portable manner, they have become the ubiquitous power source to feed portable electronics.11 Portable battery’s value chain presents environmental and ethical challenges that are similar to those facing electric and electronic equipment. Most of the available energy storage technologies still rely on hazardous compounds, such as heavy metals and/or toxic chemicals (e.g., lithium, cadmium, manganese, copper, zinc, cobalt, etc.), which are scarce and localized in specific regions in the world.12,13 In addition to being highly detrimental to the natural ecosystems,14–18 the dependence on these strategic locations is associated with geopolitical conflicts,19 hazardous working conditions20,21 and unethical supply chains.22–25
Also similarly to WEEE, the End of Life (EoL) of battery technology has been identified as one of the hot spots of its life cycle. Despite the great advances performed through environmental policies and governmental efforts covering this aspect, recycling rates through the specified waste stream for portable batteries are hardly met.26 In 2020, 220 kilotons of portable batteries were sold in EU, but the collection rate barely reached 47%.27 As a consequence, the majority of the discarded units are being irresponsibly handled, contributing to the WEEE informal management flows and ending up in unregulated recycling places. From a global perspective, there is a gap in available data on the environmental impacts of the portable batteries’ EoL scenarios, which evidences the lack of formally documented units.
Sustainable technological development
For portable batteries to stop contributing to environmental damage, and become an example for sustainable technological development, it is crucial to change the way the battery value chain is approached. In a nutshell, the actual life cycle of portable batteries still follows an obsolete linear economy model. Batteries depend on scarce non-renewable materials that are extracted by intrusive methods.28,29 Their fabrication stage takes place in centralized facilities (mostly located in Asia), generating a necessity for long-distance battery distribution around the world. Furthermore, portable battery fabrication is usually based on standardized formats. Then, electronic product developers usually decide the battery format for their application on the basis of cost rather than optimal energy requirements. As a result, batteries are often oversized for their actual energy demand and discarded after their use without being completely depleted (sometimes almost fully charged). Finally, during their EoL, if they are collected for recycling, their complex encapsulation together with the hazardous nature of the materials they are made of, make the recycling process highly energy-consuming (conventional portable battery recycling processes focus on metal recovery, being the most implemented hydrometallurgical, pyrometallurgical and thermal treatment).30 Moreover, due to the lack of local management and e-waste recycling infrastructure, battery waste is sometimes exported back again.
In this regard, the new European battery directive has become the first European legislation developed under a holistic approach establishing requirements for sustainable battery development throughout their complete life cycle.26,31 The regulation specifically tackled the minimization of scarce materials, while simultaneously presenting the requirement to strictly improve the recycling rates. The directive aims to endow European Union with self-sufficiency in the energy sector, and hence it has been mostly intended for sectors such as e-mobility and stationary energy storage systems. Such policies sometimes are hard to directly be applied to portable battery formats – due to the diverse variety of use case scenarios, their ubiquity, and that the success of the strategies depends primarily on the end-user self-responsibility.
In the portable power sources research field, from materials screening to format standardization, the main efforts have been devoted to increasing power output, achieving higher energy densities, and in the case of secondary batteries, enhancing cyclability. However, this excessive attention given to battery performance has overshadowed other indicators that set the sustainability of the battery value chain across technical, environmental, economic, and social dimensions.
Ecodesign principles to re-think the portable battery life-cycle
In this scenario, we are exploring alternative perspectives to tackle sustainability for portable batteries. Our research approach is based on the use of ecodesign principles to re-think the portable battery life-cycle under an integrated vision coupled with a strong refocusing of priorities.32,33 Profoundly inspired by the debates around the economy of growth, from the battery conception and design we take into account indicators such as materials toxicity, ethical supply chain or efficient end-of-life, to shape a sustainability ceiling.34–37 While, the power source performance in terms of power, operation time, or competitive manufacturing is set as a minimal requirement that defines the system foundation. By bringing together both boundaries, a safe and sustainable space for portable battery development emerges. Then, through careful device design and advocating for a ‘fit-to-purpose’ approach, we try to keep the battery life cycle within this safe space (Figure 1). To do so, the development of such batteries is paired with the application value chain, in such a manner that even the power source end-of-life is redefined according to the use-case scenario. Environmental sustainability is placed as a core priority to guide the batteries’ conception and implementation, assessing the impact from materials to end-of-life based on standardized methodologies.
We have recently introduced this rationale for sustainable portable battery development,38 after reporting several examples of ecodesigned power sources over the years. For instance, a paper-based battery in a lateral flow assay format intended to power portable diagnostic devices.39 These liquid-activated batteries can be fabricated under the same procedures used in the rapid test industry and have shown the ability to power the most relevant features needed in portable medical devices, such as sensors, displays, wireless communications or heating. Furthermore, alternative battery end-of-life scenarios have been explored, such as paper/cardboard recycling to power applications in smart packaging or biodegradation and compostability for applications in environmental monitoring or precision agriculture.40–42 These later batteries, made with organic materials, imitate the mechanisms of fluid transport in plants and are able to power sensing devices that measure parameters of interest in the soil and the environment; and then send the data by wireless communications. Their capability to undergo biodegradation was assessed experimentally using standardized tests, ensuring their safety disposal at the end of their lifecycle.
The proposed approach aims to spark the debate about portable battery lifecycle redefinition, to evolve towards a sustainable technological development model under an environmentally conscious framework.
Authors:
Marina Navarro-Segarra,a Mikel Undagoitia,a Carles Tortosa,a Andrea Ugalde,a Juan Pablo Esquivel a,b
a BCMaterials, Basque Centre for Materials, Applications and Nanostructures, UPV/EHU Science Park, 48940 Leioa, Spain
b IKERBASQUE, Basque Foundation for Science, 48009 Bilbao, Spain
Acknowledgements
The authors acknowledge financial support from projects EDNA (MCIN/AIE, PID2022-139250OB-C31) and BIDEKO (PLEC2021-007801), funded by MCIN/AEI/10.13039/501100011033 and European Union ‘‘NextGenerationEU/PRTR’’.
References
1. Feroz, A. K., Zo, H. & Chiravuri, A. Digital transformation and environmental sustainability: A review and research agenda. Sustainability 13, 1–20 (2021).
2. World Economic Forum. Digital Transformation – Reports – World Economic Forum. World Economic Forum (2017). at https://reports.weforum.org/digital-transformation/understanding-the-impact-of-digitalization-on-society/
3. WHO. Soaring e-waste affects the health of millions of children, WHO warns. World Health Organization 1–2 (2021). at https://www.who.int/news/item/15-06-2021-soaring-e-waste-affects-the-health-of-millions-of-children-who-warns
4. Forti, V., Baldé, C. P., Kuehr, R. & Bel, G. The Global E-waste Monitor 2020. (2020). at http://ewastemonitor.info/
5. World Health Organization (WHO). Electronic waste (e-waste). (2023). at https://www.who.int/news-room/fact-sheets/detail/electronic-waste-(e-waste)
6. Pérez-Belis, V., Bovea, M. D. & Ibáñez-Forés, V. An in-depth literature review of the waste electrical and electronic equipment context: Trends and evolution. Waste Management and Research 33, 3–29 Preprint at https://doi.org/10.1177/0734242X14557382 (2015)
7. World Economic Forum. The world’s e-waste is a huge problem. It’s also a golden opportunity. at https://www.weforum.org/agenda/2019/01/how-a-circular-approach-can-turn-e-waste-into-a-golden-opportunity/
8. Puckett, J., Brandt, C. & Palmer, H. Holes in the Circular Economy: WEEE Leakage from Europe. A Report of the e-Trash Transparency Project. A Report of the e-Trash Transparency Project 120 (2018). at
9. Juan Ignacio Incardona| Planeta Furturo | EL PAÍS. El antiguo gran basurero electrónico de Ghana se resiste a morir . (2023). at https://elpais.com/planeta-futuro/2023-05-11/jugarse-la-vida-en-el-gran-cementerio-electronico-de-ghana-inundado-con-la-basura-del-norte.html
10. Holuszko, M. E., Kumar, A. & Espinosa, D. C. R. Electronic waste : recycling and reprocessing for a sustainable future. (2021).
11. Word Economic Forum. A New Circular Vision for Electronics, Time for a Global Reboot. (2019). at https://www.weforum.org/publications/a-new-circular-vision-for-electronics-time-for-a-global-reboot/
12. Koyamparambath, A., Santillán-Saldivar, J., McLellan, B. & Sonnemann, G. Supply risk evolution of raw materials for batteries and fossil fuels for selected OECD countries (2000–2018). Resources Policy 75, (2022).
13. Intenational Energy Agency (IEA). The Role of Critical Minerals in Clean Energy Transitions. (2021). at https://www.iea.org/reports/the-role-of-critical-minerals-in-clean-energy-transitions/executive-summary
14. Opray, M. Nickel mining: the hidden environmental cost of electric cars. (2017). at https://www.theguardian.com/sustainable-business/2017/aug/24/nickel-mining-hidden-environmental-cost-electric-cars-batteries
15. El impacto de una de las minas de cobre más grandes del mundo. (2019). at 16. Maxwell, A., M. Balcázar, R. & Barandiarán, J. Exhausted: How We Can Stop Lithium Mining from Depleting Water Resources, Draining Wetlands, and Harming Communities in South America. (2022). at https://www.nrdc.org/resources/exhausted-how-we-can-stop-lithium-mining-depleting-water-resources-draining-wetlands-and 17. Geenfield, N. Lithium Mining Is Leaving Chile’s Indigenous Communities High and Dry (Literally). Preprint at https://www.nrdc.org/stories/lithium-mining-leaving-chiles-indigenous-communities-high-and-dry-literally (2022) 18. Dave Sherwood. A water fight in Chile’s Atacama raises questions over lithium mining. Preprint at (2018) 19. D. Olmo, G. Chalcobamba, la montaña rica en cobre que enfrenta a una gran minera china con unos jóvenes peruanos. (2022). at 20. The Guardian. Pollution causing birth defects in children of DRC cobalt miners – study. (2020). at https://www.theguardian.com/global-development/2020/may/06/pollution-causing-birth-defects-in-children-of-drc-cobalt-miners-study 21. Amnesty International & Afrewatch. THIS IS WHAT WE DIE FOR”: HUMAN RIGHTS ABUSES IN THE DEMOCRATIC REPUBLIC OF THE CONGO POWER THE GLOBAL TRADE IN COBALT. (2016). at https://www.amnesty.org/en/wp-content/uploads/2021/05/AFR6231832016ENGLISH.pdf 22. Kang, D. H. P., Chen, M. & Ogunseitan, O. A. Potential Environmental and Human Health Impacts of Rechargeable Lithium Batteries in Electronic Waste. Environ Sci Technol 47, 5495–5503 (2013). 23. Sharma, S. S. & Manthiram, A. Towards more environmentally and socially responsible batteries. Energy Environ Sci 13, 4087–4097 (2020). 24. Baumann-Pauly, D. Cobalt Mining in the Democratic Republic of the Congo: Addressing Root Causes of Human Rights Abuses. Preprint at https://static1.squarespace.com/static/5b6df958f8370af3217d4178/t/63e2dc7bad25b047da9100d7/1675811964954/NYU+CBHR+Cobalt+Mining_FINAL+Feb7.pdf (2023) 25. Amnesty International. República Democrática del Congo: La minería industrial de cobalto y cobre para baterías recargables está dando lugar a graves abusos contra los derechos humanos. (2023). at https://www.amnesty.org/es/latest/news/2023/09/drc-cobalt-and-copper-mining-for-batteries-leading-to-human-rights-abuses/ 26. European parliament and the Council of the European Union. REGULATION (EU) 2023/1542 . (2023). at https://eur-lex.europa.eu/legal-content/EN/TXT/PDF/?uri=CELEX:32023R1542 27. Eurostat. Recycling of batteries and accumulators. eurostat 1–14 (2020). at https://ec.europa.eu/eurostat/statistics-explained/index.php?title=Waste_statistics_-_recycling_of_batteries_and_accumulators&stable=0#Sales_and_collection_of_portable_batteries_and_accumulators 28. ECa. European Commission, Critical Raw Materials for Strategic Technologies and Sectors in the EU. A Foresight Study (2020). doi:10.2873/865242 29. Liu, C., Lin, J., Cao, H., Zhang, Y. & Sun, Z. Recycling of spent lithium-ion batteries in view of lithium recovery: A critical review. J Clean Prod 228, 801–813 (2019). 30. Suchi Gopal. Recycling of Lithium Batteries and GHG Emissions. (2023). at https://floodlightinvest.com/recycling-of-lithium-batteries-and-ghg-emissions/ 31. Commission, E. & Innovation, D.-G. for R. and. European Green Deal – Research & innovation call. (Publications Office of the European Union, 2021). doi:doi/10.2777/33415 32. Raworth, K. A safe and just space for humanity: can we live within the doughnut? Oxfam Discussion Paper. State of the World 2003: Progress Towards a Sustainable Society: 20th Edition 1–240 (2012). at www.oxfam.org/grow 33. O’Neill, D. W., Fanning, A. L., Lamb, W. F. & Steinberger, J. K. A good life for all within planetary boundaries. Nat Sustain 1, 88–95 (2018). 34. Fischer-Kowalski, M. & K. Steinberger, J. in Handbook on Growth and Sustainability (eds. Peter A. Victor & Brett Dolter) (Edward Elgar Publishing, 2017). 35. Hickel, J., Kallis, G., Jackson, T., O’Neill, D. W., Schor, J. B., Steinberger, J. K., Victor, P. A. & Ürge-Vorsatz, D. Degrowth can work — here’s how science can help. Nature 612, 400–403 (2022). 36. Richardson, K., Steffen, W., Lucht, W., Bendtsen, J., Cornell, S. E., Donges, J. F., Drüke, M., Fetzer, I., Bala, G., von Bloh, W., Feulner, G., Fiedler, S., Gerten, D., Gleeson, T., Hofmann, M., Huiskamp, W., Kummu, M., Mohan, C., Nogués-Bravo, D., Petri, S., Porkka, M., Rahmstorf, S., Schaphoff, S., Thonicke, K., Tobian, A., Virkki, V., Wang-Erlandsson, L., Weber, L. & Rockström, J. Earth beyond six of nine planetary boundaries. Sci Adv 9, (2023). 37. van den Bergh, J. C. J. M. Environment versus growth — A criticism of “degrowth” and a plea for “a-growth”. Ecological Economics 70, 881–890 (2011). 38. Navarro-Segarra, M. & Esquivel, J. P. in Sustainable Energy Storage in the Scope of Circular Economy (2023). 39. Esquivel, J. P., Del Campo, F. J., Gómez De La Fuente, J. L., Rojas, S. & Sabaté, N. Microfluidic fuel cells on paper: meeting the power needs of next generation lateral flow devices. Energy Environ Sci 7, 1744–1749 (2014). 40. Navarro-Segarra, M., Tortosa, C., Ruiz-Díez, C., Desmaële, D., Gea, T., Barrena, R., Sabaté, N. & Esquivel, J. P. A plant-like battery: a biodegradable power source ecodesigned for precision agriculture. Energy Environ Sci 15, 2900–2915 (2022). 41. Esquivel, J. P., Alday, P., Ibrahim, O. A., Fernández, B., Kjeang, E. & Sabaté, N. A Metal‐Free and Biotically Degradable Battery for Portable Single‐Use Applications. Adv Energy Mater 7, (2017). 42. Navarro-Segarra, M. Primary batteries paradigm redefinition within the environmental planetary boundaries. TDX (Tesis Doctorals en Xarxa) (2022). at https://www.tdx.cat/handle/10803/675084