Different surface structures affect catalytic activity of silver
Catalysis in industry is often driven by empirical investigations and, eventually, by unexpected discoveries. Frequently, the scientific understanding of the process takes much longer to develop. Ideally, it would be preferable to reverse this timeline, where scientific insights precede application. Nonetheless, even when the scientific explanation takes more time, a deep understanding of the underlying processes can lead to greater innovation and efficiency.
In new study 1, a team of scientists addresses such a catalytic process. For nearly 100 years, silver (Ag) has been used as a catalyst in several important reactions: (i) the conversion of methanol to formaldehyde, and (ii) the epoxidation of ethylene and propylene to ethylene oxide (EO) and propylene oxide. The global production of these products reaches nearly 50 million tons annually, i.e., such reactions and the catalyst are very relevant. In industry, oxygen and either methanol, ethylene, or propylene are introduced into reactors under high pressure and temperature conditions, leading to the oxidation of Ag, which serves as the catalyst.
In particular, the ethylene-to-EO reaction has attracted significant scientific interest. Industrially, this Ag-catalyzed reaction, assisted by promoters and mediators, achieves selectivities above 90%. Here, selectivity refers to the conversion towards EO and the avoidance of undesired complete oxidation of ethylene to carbon dioxide and water. Despite extensive research over the past 40 years, a clear understanding of the process remained elusive. However, five years ago, an international consortium of European scientists made a surprising discovery: the desired conversion of ethylene to EO is not facilitated by silver alone, but requires trace sulfur impurities. This was unexpected, as sulfur is not considered in the industrial process. In the recent study led by the San Sebastian-based scientists, not only do they confirm this observation, but they also propose, using their curved crystal approach, specific surface structures that are more favorable for sulfur accumulation, thereby providing deeper insights into the process.
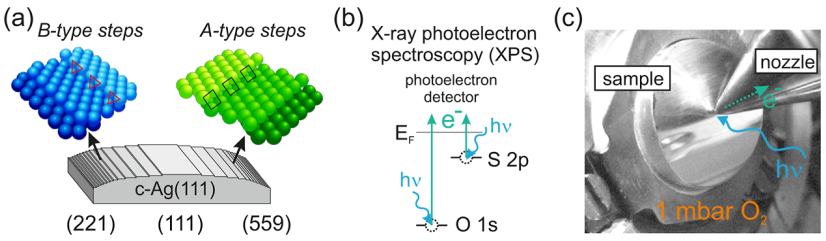
To achieve this, experiments were conducted at the ALBA synchrotron radiation facility in Barcelona. The curved single crystal, as depicted in Fig. 1(a), was exposed to 1 mbar of oxygen at temperatures near those used for ethylene to ethylene oxide (EO) conversion. Under these conditions, photoemission spectroscopy data were analyzed as a function of various crystal positions, as shown in Figs. 1(b) and 1(c). The unique curved crystal approach, a specialty of the researcher, allows for the identification of areas on the crystal where sulfur accumulation is either minimal or maximal, as illustrated in Figure 2.
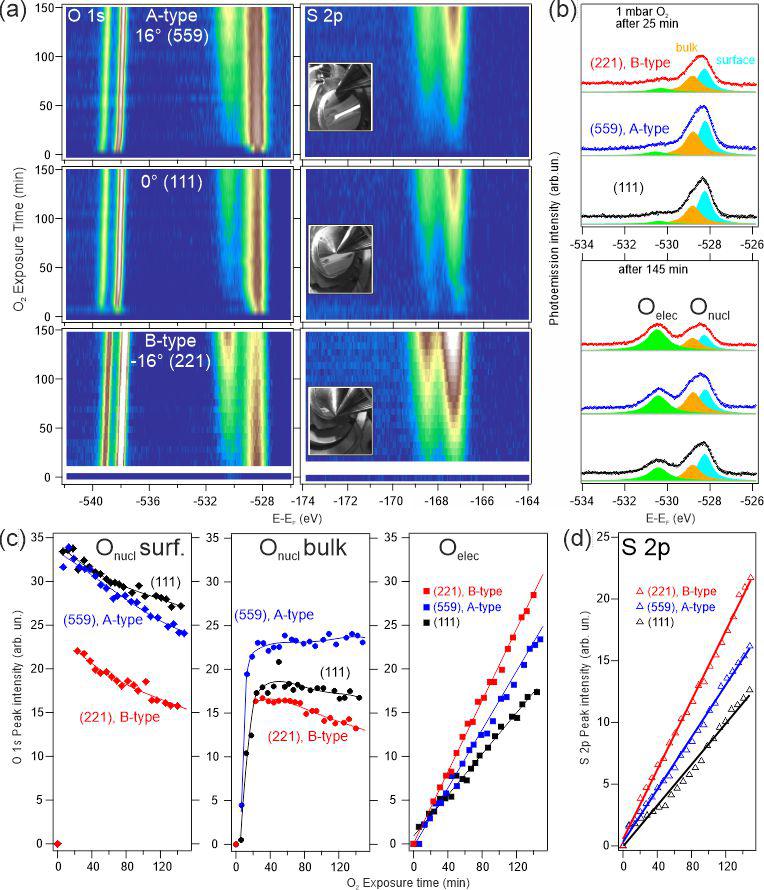
The core level emission spectra of oxygen reveal distinct peaks at higher binding energies, indicating the presence of surface species responsible for catalytic activity. Notably, the electrophilic oxygen peak around 530.2 eV increases in tandem with the sulfur 2p peak emission, confirming sulfur dioxide accumulation on the surface. In contrast, the peak for pure silver oxide at 528 eV decreases over time. Analysis of the results from three key crystal positions reveals that the flat (111) surface accumulates the least sulfur, while stepped regions, particularly B-type stepped surfaces, show significantly higher sulfur concentrations—up to twice as much. These findings suggest that different surface structures affect catalytic activity under reaction conditions, providing valuable insights for designing more efficient catalytic materials.
Author: Frederik Schiller is a tenured scientist at the Nanophysics Lab of the Materials Physics Center (CFM-CSIC) and a DIPC Associate
References
- Frederik Schiller, Khadiza Ali, Anna A. Makarova, Sabine V. Auras, Fernando García-Martínez, Alaa Mohammed Idris Bakhit, Rodrigo Castrillo Bodero, Ignacio J. Villar-García, J. Enrique Ortega, Virginia Pérez-Dieste (2024) Near-Ambient Pressure Oxidation of Silver in the Presence of Steps: Electrophilic Oxygen and Sulfur Impurities ACS Catalysis doi: 10.1021/acscatal.4c02985 ↩