Unlocking magnetism in nanographenes: The role of oxyradicals and molecular design
Nanotechnology is like a playground for scientists, where they tinker with materials at the scale of atoms to create new tools and devices with extraordinary properties. One of the most exciting areas in this field involves nanographenes, which are tiny pieces of graphene—a single layer of carbon atoms arranged in a honeycomb pattern, known for its strength and ability to conduct electricity. A groundbreaking study 1 dives into how these nanographenes can be designed to have magnetic properties, opening doors to future technologies like super-fast computers or advanced sensors.
Nanographenes and oxyradicals
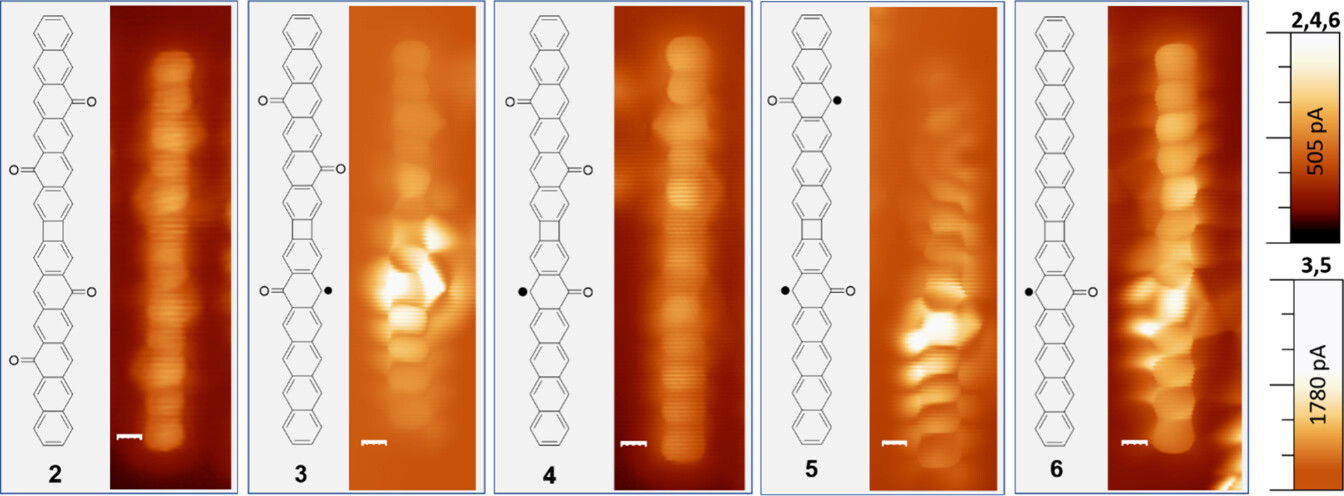
Picture graphene as a vast, flat sheet of carbon atoms linked in a hexagonal grid, like a microscopic chicken wire. Nanographenes are small fragments of this sheet, carefully crafted into specific shapes. Their properties depend on their size, shape, and the arrangement of their edges, which can influence how electrons move within them. In this study, scientists created a special type of nanographene called linear hexacene dimers, which are like two long chains of carbon rings joined together by a small, four-membered carbon ring. These molecules are modified with oxygen-containing groups called carbonyls, which give rise to something called oxyradicals.
Oxyradicals are fascinating because they’re molecules with an unpaired electron, making them chemically reactive and, importantly, magnetic. This magnetism comes from the way electrons are arranged in the molecule’s structure, a property known as π-magnetism because it involves the electrons in the carbon’s π-orbitals, which are part of the molecule’s extended electron cloud. Unlike traditional magnets made from metals like iron, these carbon-based magnets are lightweight and could be used in cutting-edge applications like spintronics, where electron spin is used to process information.
Crafting molecules with precision
To create these nanographenes, the researchers used a technique called on-surface synthesis, which is like assembling a puzzle on a perfectly smooth table. They started with a molecule called an ortho-dibrominated hexacene derivative, decorated with bromine atoms and epoxy groups (oxygen bridges) to make it stable and easier to work with. This molecule is placed on a gold surface, which acts like a clean canvas. When heated to around 230°C, the bromine atoms break off, allowing the molecules to link up through new carbon-carbon bonds in a process called cycloaddition. This forms the hexacene dimers with a central four-membered ring.
The epoxy groups also transform during this heating process. Most of them turn into carbonyl groups, which add an extra electron to the molecule’s π-system, creating an oxyradical. Sometimes, though, the epoxy groups completely detach, leaving a simpler carbon structure behind. By carefully controlling this process, the scientists created a family of nanographenes with one to four carbonyl groups, each with different magnetic properties depending on where these groups are placed.
The role of magnetism in nanographenes
Magnetism at this tiny scale is a big deal because it could change how we design electronics. In traditional computers, data is stored as electrical charges, but in spintronics, data could be stored and processed using the spin of electrons, which is like a tiny magnetic arrow pointing up or down. The nanographenes in this study can be magnetic because of their oxyradicals, which create unpaired electrons. The researchers found that the magnetic behaviour depends on where the carbonyl groups are located on the molecule.
The carbon atoms in these nanographenes form a bipartite lattice, meaning they can be divided into two groups where each atom only connects to atoms in the other group. This structure is key to understanding their magnetism. When two carbonyl groups are on the same side of a hexacene segment, their radicals (unpaired electrons) sit on the same group of atoms and can’t cancel each other out, leading to a magnetic state. If they’re on opposite sides, the radicals cancel each other, forming a non-magnetic molecule. The study shows how small changes in the molecule’s design can switch its magnetism on or off, like flipping a tiny magnetic switch.
How charge transfer affects magnetism
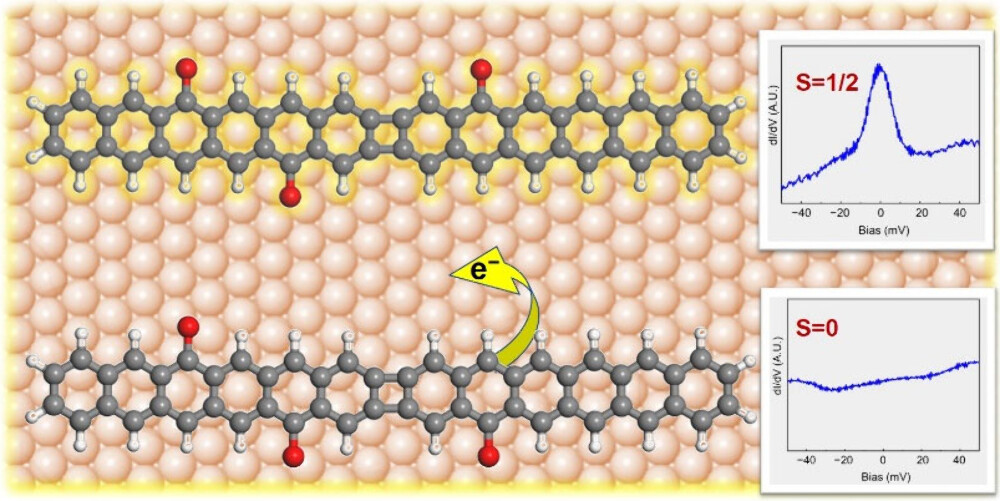
Another critical factor in this research is how these nanographenes interact with the gold surface they’re placed on. Electrons can sometimes move from the molecule to the gold, a process called charge transfer. Whether this happens depends on the molecule’s ionization energy—the energy needed to remove an electron. Molecules with more carbonyl groups have higher ionization energies because the oxygen atoms pull electron density away from the carbon framework, making it harder for electrons to leave.
The researchers used computer simulations to calculate the ionization energies of their nanographenes, finding that those with fewer carbonyl groups (like one or two) are more likely to transfer an electron to the gold surface, which can suppress their magnetism. In contrast, molecules with three or four carbonyl groups hold onto their electrons better, preserving their magnetic properties. This interplay between the molecule’s structure and its interaction with the surface is crucial for designing nanographenes with the desired magnetic behaviour.
Peering into the molecules
To study these tiny structures, the scientists used a technique called scanning tunneling microscopy (STM), which is like an ultra-precise camera that can capture images of individual atoms. By scanning a tiny needle over the gold surface, they could see the exact shape of each nanographene and confirm that the synthesis worked as planned. They also measured the electronic properties of the molecules using differential conductance spectra, which reveal how electrons are distributed in the molecule’s energy levels. These measurements, combined with computer simulations, showed how the position of carbonyl groups affects the molecule’s electronic and magnetic properties.
A glimpse into the future
This research is a big step forward because it shows how to control magnetism at the nanoscale by tweaking the design of carbon-based molecules. These nanographenes could be used in spintronics to create faster, more efficient devices, or in quantum computing, where magnetic properties could help store quantum information. Because they’re made of carbon, they’re also lightweight and potentially safe for use in medical applications, like targeted drug delivery or advanced sensors.
However, there are challenges to overcome. On-surface synthesis requires highly controlled environments, which are expensive and hard to scale up. The stability of these nanographenes outside the lab also needs more study. Still, the ability to fine-tune their properties by adjusting the number and position of carbonyl groups is a powerful tool for scientists, paving the way for new materials with tailored functions.
Author: César Tomé López is a science writer and the editor of Mapping Ignorance
Disclaimer: Parts of this article may have been copied verbatim or almost verbatim from the referenced research paper/s.
References
- Tao Wang, Sergio Salaverría, Fernando Aguilar-Galindo, Javier Besteiro-Sáez, Luis M. Mateo, Paula Angulo-Portugal, Jonathan Rodríguez-Fernández, Dolores Pérez, Martina Corso, Diego Peña & Dimas G. de Oteyza (2025) Relating Radical Delocalization, Charge Transfer, and Magnetic Ground State in Acene-Derived Oxyradicals Nano Letters doi: 10.1021/acs.nanolett.5c00263 ↩