Mechanochemistry of nanographenes
Modern organic industrial chemistry started when William Henry Perkin serendipitously synthesized mauveine in 1856 while he was attempting the total synthesis of quinine. Since then, thousands of new organic products have been created in the laboratory for industrial purposes. Among them, in 1913, an orange-red pigment was found, first known as Vat Orange 3 dye, it became more popular as a pigment, Pigment Red 168. Now, more than a century later, a team of researchers, including Jon Matxain and David Casanova from DIPC and UPV/EHU, use 1 this pigment as the base for a new breakthrough in the mechanochemistry of nanographenes.
Graphene, as you probably know, is a transparent two-dimensional sheet of carbon atoms arranged in hexagons. But it is not a molecule, it is a sheet, a single-layer of graphite, one of the allotropes of carbon. Nanographenes are the molecular version of graphene; we can see them as finite forms of the theoretically infinite sheet that is graphene. A nanographene diradical is a nanographene with two group of atoms where there is an unpaired valence electron.
The study of nanographene diradicals has been the subject of intense research because of the outstanding spin and electronic properties of graphene and the opportunity to investigate fundamental aspects of chemical bonding. Singlet diradical molecules are characterized by unique optoelectronic properties of interest to nonlinear optics, spintronics, and organic photovoltaics.
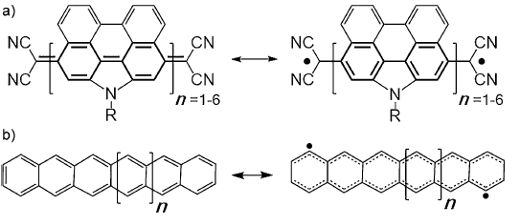
Several strategies for attaining such biradicaloid molecules with varying degrees of diradical character have been developed (see Figure 1). Such strategies include: 1) accumulation of proaromatic rings in oligomeric structures; 2) construction of antiaromatic molecules; and 3) design of nanographenes with appropriate zig–zag edge states (that is, metallic or half-metallic).
However, from the practical point of view stability is key to enable the study of the unique properties of diradicals. In cases 1) and 2), smaller oligomers are highly stable molecules due to a full bonding electronic configuration, but diradicality is only observed in longer oligomers. The preparation of these long systems often requires tedious synthesis and the resulting oligomers are not that stable. Here is when dye-related chemistry gets in.
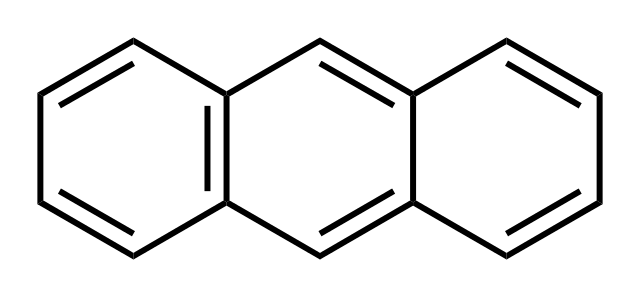
Anthracene, an aromatic hydrocarbon with three fused rings (see Figure 2), is obtained by the distillation of crude oil and it is mainly used in the manufacture of dyes. The anthracene substructure has been utilized in the design of biradicaloid molecules before.
In pursuit of a robust polycyclic framework to accommodate stable diradicals, the researchers used the low-cost and commercially available Pigment Red 168, 4,10-dibromoanthanthrone, for the preparation and characterization of a new “nanographene”.
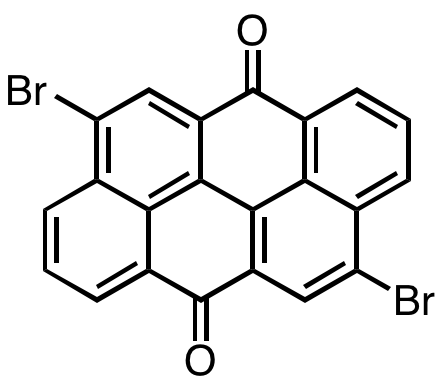
While the anthanthrone core can be viewed as two anthracene ‘parts’ with lateral functionalization (see Figure 3) , its chemical and physical properties stand in sharp contrast with the parent anthracene subunit; anthanthrone exhibits remarkable stability because of extended delocalization.
The researchers hypothesized that the general target structure shown in Figure 4 would possess an accessible diradical state upon core planarization, as this would result in the release of steric strain along with the formation of two perpendicular π systems.
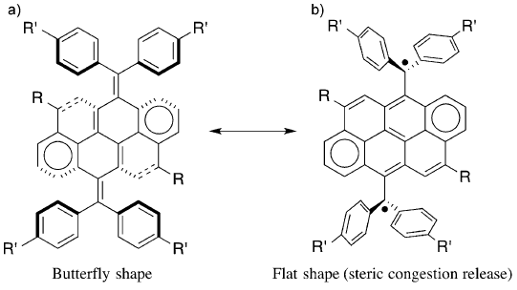
This means that this system would provide a rare example of a closed-shell to diradical transition fueled exclusively by strain release. Thus, it would not matter how the strain release is achieved. Of course, the planarization of the core can be attained in a typical physicochemical way: in solution at moderate temperatures. But, the researchers show, that planarization is also achieved in a purely mechanical fashion, that is, by the application of mild pressure in the solid state. The molecule, after planarization in the solid state, forms a kinetically trapped diradical, which the scientists characterized by Raman and EPR spectroscopies in combination with quantum chemical calculations.
This rare example of π bond breaking by mechanochemistry opens the way to the generation of diradicaloid species with accessible high spin states from robust quinoidal structures.
Author: César Tomé López is a science writer and the editor of Mapping Ignorance.
References
- Maude Desroches, Paula Mayorga Burrezo, Joël Boismenu-Lavoie, Miriam Peña Álvarez, Carlos J. Gómez-García, Jon M. Matxain, David Casanova, Jean-François Morin, and Juan Casado (2017) Breaking Bonds and Forming Nanographene Diradicals with Pressure Angew. Chem. Int. Ed. doi: 10.1002/anie.201708740 ↩