Ice Cube, the frozen observatory
Deep in the ice below the Amundsen-Scott South Pole Station, in Antartica, lies one of the great works that our modern civilization has produced. A kilometer and a half under the frozen surface there is a very special place for science and discovery. Darkness surrounds it but it’s able to see the entire cosmos. The Ice Cube Neutrino Observatory, with the size of one cubic kilometer, is one of the most carefully crafted, yet massive in scale, science tools that our species has ever produced. This device -that’s one way to call it- is made to see neutrinos, maybe the most elusive particle that we ever discovered, a particle that holds the key to understanding the origin of the universe itself.
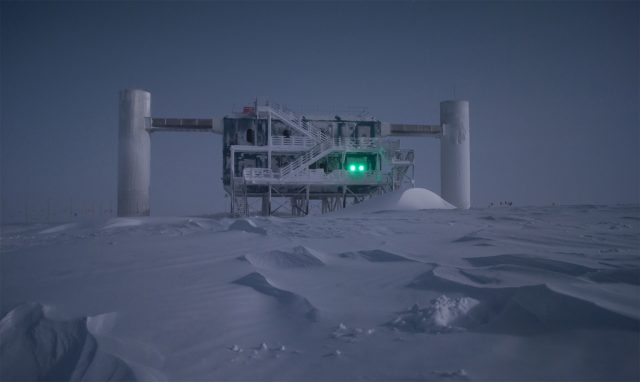
How wonderful is it that to understand the most subtle physics of the cosmos we have to go to the most remote places. This observatory does not see visible light. It detects something called Cherenkov radiation, a haunting blue light that particles traveling faster than light emit. Of course, nothing travels faster than light in the vacuum, but, similarly to the sound that travels faster on water and slower on air, light travel at different speeds in different mediums. This effect is especially notable in water (or ice), where some radiation particles going at great speeds, almost touching the speed of light, can travel faster than the light travels through the water. A quick YouTube search with the keywords “Nuclear Reactor Startup” reveals this effect. When a reactor, usually sunk on water, turns on, some of the radiation produced travels to the water at near-light speeds, producing this blue light. One can think of a plane traveling faster than the sound, we have all seen those wonderful photos of shock cones around a jet, produced when breaking the sound barrier. This is caused by air getting compressed by the plane. Since the air cannot move faster than the speed of sound, and the plane is compressing it at that very high speed, a high-pressure zone is created generating the cone. Cherenkov radiation works in a similar way, the particle traveling faster than light in the medium emits light that gets “packed” in a shock cone. This cone, if one does the numbers, will shine in that mystique blue. And the amount of blue light produced by the traveling particle is proportional to the speed of it. So, the faster the particle moves, the more intense that blue will be.
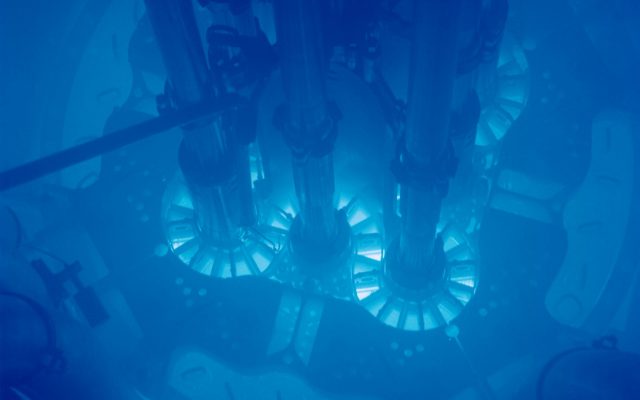
Neutrinos are that fast, but they are not charged so they cannot emit any light. In fact, Neutrinos are really really hard to get. They are extremely light, so light that no one knows, yet, their mass. They are also neutral, forbidding them to interact with anything else in a common way. They can only “hit” something through the Weak force, the subtlest of the four natural forces. Billions of Neutrinos are cutting through ourselves every second, coming from the sun, and nobody feels anything. They pose no harm, they rarely interact with anything. But when they decide to, they transfer all their energy to the unlucky atom that happened to be in their trajectory, generating all sort of charged particles from the shreds of that atom. Those particles are ejected so fast that can produce Cherenkov radiation in the water. And, simply put, that’s how Ice Cube “sees” Neutrinos.
Ice Cube consists on, so far, 86 three kilometer strings with light detectors spaced every few meters. Each of those strings is submerged into the ice, in a deep drilled hole, and each string is spaced a few dozens of meters from the next one in a hexagonal array. If a charged particle, a sub-product of a neutrino interaction with the ice, traverses the volume covered with the detectors producing Cherenkov light, the detectors can measure the timing of that shock wave hitting the light sensors. With that information, the energy and trajectory of the incoming neutrino can be inferred. There are other similar approaches to neutrino detection around the world, such as Super-Kamiokande in Japan, lying in an underground artificial cavern filled with water with the detectors in the walls, floor and ceiling of it, but none of the scale of Ice Cube.
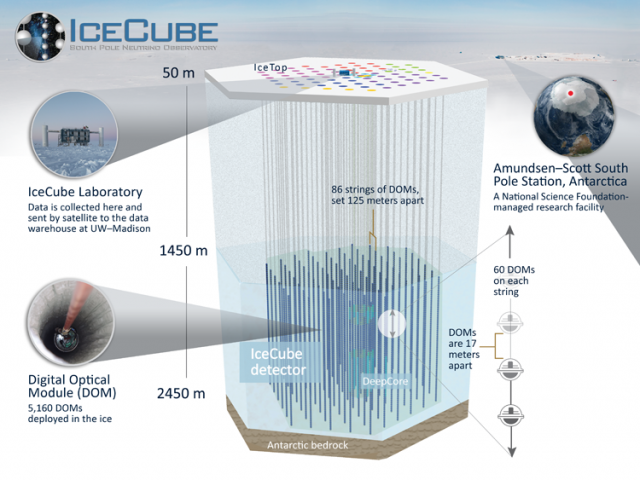
The possibilities are endless here. Neutrinos are emitted everywhere in the galaxy. Our sun is an overwhelming source of neutrinos, making it a great object of study, for instance. Other source are the neutrinos produced as the sub products of a cosmic ray hitting some atom in the atmosphere. A shower of particles is produced in this scenario, one of them being neutrinos. This could help to unveil one of the mysteries surrounding Neutrinos: they oscillate. There are three possible types of neutrinos, electronic, muon and tau, each having its own different mass. And a neutrino can change, or oscillate, from one type to another. Also, there is the hierarchy problem. The actual masses of the neutrino types are unknown, but the relative mass difference between them are. Knowing their absolute masses would give us a hint on the birth of the universe, because that might explain why there is not antimatter around, a paradox known as the matter-antimatter asymmetry, but not even the Standard Model of physics can fully understand this mechanism (yet). Ice Cube was able to measure with great precision the difference between two of the neutrino phases using the ones produced in the atmosphere when a cosmic ray hits it 1.
Another wonderful application deals with another wonderful problem in astrophysics: gamma ray bursts. Since our technology allowed us, a lot of mysterious bursts of high energy photons, known as gamma ray bursts, has been observed hitting the earth. They come from the most distant places of the universe and they are assumed to be the sons of violent events such as supernovae or rapidly rotating high mass stars. Lasting from milliseconds to hours, they are the most energetic emissions ever recorded here on Earth. Ice Cube plays a lead role on unveiling the sources of these events, since a supernova, for instance, should produce lots of neutrinos, and they should hit earth a few minutes earlier than the gamma ray itself (since the light must cut through the exploding star, slowing a little bit, and the neutrino just traverses it at nearly light speed). By measuring the number of neutrinos hitting the earth from the same direction of a posterior gamma ray burst one can deduce the nature of that event. But, no neutrino has been yet observed linked to a gamma ray burst, lowering the bar for expected neutrino flux that the theory sets, and by doing so, expanding the mystery of gamma ray bursts 2.
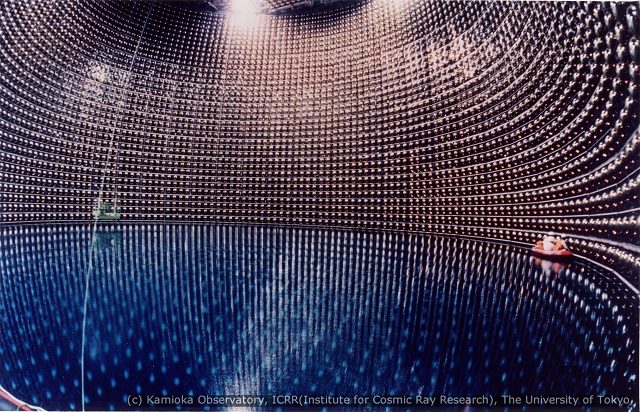
Also, this brings us to another application: The Supernova Early Warning System. This is a joint collaboration of worldwide detectors which Ice Cube forms part of, focused in the early detection of supernova events, within our galaxy and beyond. As said before, there is a slight temporal difference between the neutrinos and the light emitted in a supernova. Neutrinos can hit the earth minutes or hours before the light does, giving us a head start to detect this light. In the event of an early warning, all the telescopes from earth would point to the neutrino source in a hurry, trying to be ready for the visible supernova explosion, and gathering the data from one of the holy grails of Astrophysics: a live galactic Supernova if it is the case. Ice Cube does not fit well the requirements for extra-galactic supernova neutrinos, but it might be able to detect those emitted from an event within our galaxy. Those events are quite common on the universe, but in our galaxy there can be hundreds of years without a single one. Astrophysics are quite impatient to see one with our modern equipment. The last recorded event was first observed by Chinese astronomers around 1054 of our age, and today we know it as the Crab nebula, a quite illustrative image of what a Supernova leaves behind.
Image: IceCube eveng.jpg | A reconstruction of an event in the IceCube detector. The vertical lines are the strings where the light detectors are mounted, and the diagonal one is the inferred trajectory of the detected particle. In color, the particular detectors that saw the Cherenkov light with its relative intensity. Credit: The IceCube Collaboration
Ice Cube is a promise of a new age in astrophysics. The last application that I’m going to talk about is Dark Matter detection. There is the hypothesis that some dark matter particles should be captured in our Earth’s core, orbiting them as a spacecraft would do in the space around Earth. Dark Matter would annihilate itself and one of the byproducts of that event would be neutrinos. An excess of neutrinos in a particular energy and direction would imply that Dark Matter is there, but just some weeks ago Ice Cube published a paper saying that, so far, they did not detect any Dark Matter-related source of neutrinos. This was quite expected, sine the sensitivity of Ice Cube for this purpose is far worse than other Dark Matter-dedicated experiments around the world that already produced that result, but it’s another example of the versatility of the experiment 3. Wonderful design and wonderful ideas leads to wonderful science.
References
- M. G. Aartsen et al. (IceCube Collaboration) (2015) Determining neutrino oscillation parameters from atmospheric muon neutrino disappearance with three years of IceCube DeepCore data Phys. Rev. D 91, 072004 DOI: 10.1103/PhysRevD.91.072004 ↩
- M. G. Aartsen et al. (IceCube Collaboration) (2015) Search for Prompt Neutrino Emission from Gamma-Ray Bursts with IceCube, The IceCube Collaboration ApJ 805 L5 DOI: 10.1088/2041-8205/805/1/L5 ↩
- M. G. Aartsen et al. (IceCube Collaboration) (2017) First search for dark matter annihilations in the Earth with the IceCube Detector Eur. Phys. J. C DOI: 10.1140/epjc/s10052-016-4582-y ↩
2 comments
[…] gauza xume bezain garrantzitsua gertatu zen munduaren txoko urrun batean: Antartidan kokatutako IceCube izeneko behatokian, zientzialariek “ikusezina” zen partikula baten arrastoa “ikusi” ahal […]
[…] nukleo atomikoekin talka egiten dutenean sortzen dira neutrino atmosferikoak. Partikula horiek IceCube behatokiari esker atzeman ahal izan dituzte. Antartikan kokatuta dagoen behatoki horrek, besteak […]