The Josephson heat interferometer
Thermodynamics is one of the oldest and more fruitful fields of science. Quantum mechanics, on the other hand, is less than one hundred years old, but it is also useful and well accepted. They can look very different. Thermodynamics treats with macroscopic systems, composed by an Avogadro number (6.023×1023) of atoms or molecules, and quantum mechanics deals with the microscopic world. Of course, both theories should be related, as the macroscopic world is composed by many microscopic systems, but this relation is difficult to formulate. There are also macroscopic quantum effects, as the one treated in this post, and when these effects are mixed with thermodynamical laws, we can only expect to have interesting and non-intuitive behaviors.
One of the principal goals of thermodynamics is to determine which processes can be performed and which ones cannot. One of the most important results in this direction is the second law of thermodynamics. It states, in Clausius’ formulation, that: “No process is possible whose sole result is the transfer of heat from a body of lower temperature to a body of higher temperature”. Bad news for free-energy refrigerators and eternal movement machines. That means that the only option to transfer heat from a cold to a hotter one is to expend some work on it, just as regular refrigerators do using electrical power.
Another classical result, this time coming from quantum mechanics, is the Josephson effect. This was proposed in the sixties 1, and it states that the electric current in a junction between two superconductors coupled by a weak link, what is sometimes called a supercurrent, depends on a property called the relative quantum phase between both parts. The most interesting conclusion, regarding the Josephson effect, is that applying an external magnetic field to the junction we can control this current. That represents a macroscopic quantum effect, and it has many applications for cutting-edge technology. The Josephson effect allows experimentalists to create Superconducting Quantum Interference Devices (SQUIDs) 2. These devices play a very important role in modern technologies, like quantum computing.
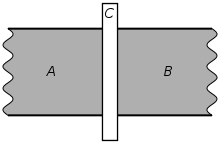
Only recently, a new type of Josephson effect has been probed in the lab, by F. Giazotto and M.J. Martínez-Pérez 3. This time, the electric current is not the matter of study. Instead of that the researchers have focused on the heat transfer between the two superconductors. The scheme of the device can be seen in Figure 2. There, superconductors at different temperatures are connected by a weak interphase. By tunnel effect electrons can jump across this interphase, creating a heat flux. The quantum phases, a magnitude that can be controlled by the magnetic field, determines the strength of this flux.
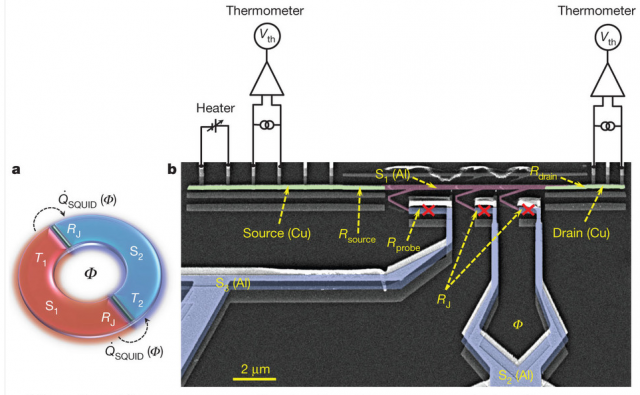
And what did they measure? Basically, by knowing the temperatures of the different parts of the device the experimenters were able to calculate the heat flux for different values of the external field. As quantum mechanics predicts, there is a strong dependence between these two magnitudes. That means that it is possible to control the heat just by the use of a magnetometer, and it can even flow from the cold superconductor to the hot one. This dependence makes the temperature of the drain (see Figure 2, right) to oscillate with the magnetic field.
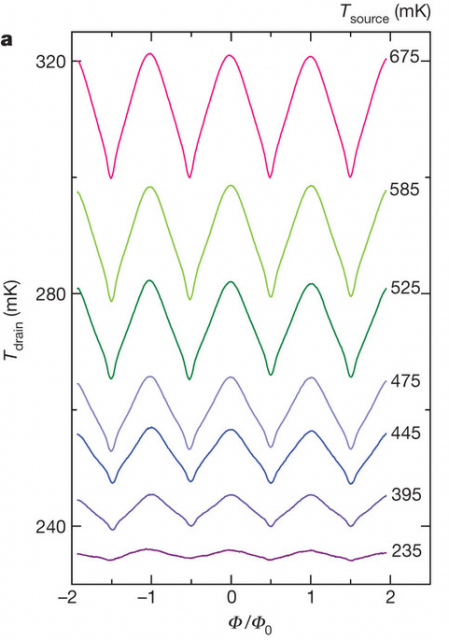
What happens with the second law of thermodynamics, then? Well, it is not violated, as expected. For understanding this one has to take into account other fluxes that appear in the complete experimental setup. In the next figure all the heat fluxes involved in the experiment are displayed.
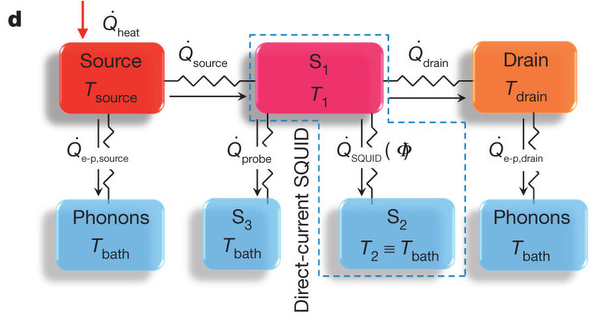
The “violation” of the second law of thermodynamic only arises if we consider just one of the currents, the one labeled in Figure 2 as the “direct-current SQUIDs”, but this approach is incorrect. This thermodynamical law can only be applied to an isolated system, so we have to consider the complete system and sum up all fluxes. By doing this it can be probed that the net flux still fulfills the second law.
So, this experiment represents an empirical demonstration of a very old quantum mechanical prediction, and a new insight into macroscopic quantum effects. From a more practical point of view, the possibility of controlling heat just with an external magnetic field can open new lines of research in applied physics. It can help us to design new technology as highly efficient refrigerators, electronic devices and, maybe, quantum computers. Only time can show how useful itwill be.
2 comments
Nice article!
however your first reference is not correct…
Physics Letters, vol. 1, Issue 7, pp.251-253
You are right, I confused Physics Letters with Physical Review Letters.