Mantle convective flow determines the depth of the ocean
The depth of the ocean is directly related to the behavior of the tectonic plates. Studies conducted so far have assumed that the sea floor depth depends solely on its age. However, these results do not match the experimental measurements.
The work developed by Adam and Vidal 1 shows that the convective flow of the underlying viscous mantle plays a strong role on the plates’ movement and, therefore, on the sea-floor depth.
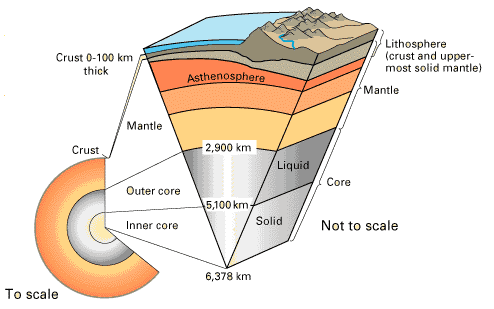
The inner structure of the Earth is divided into layers with different chemical or physical properties (Figure 1). Beneath the solid outer layer, called crust, is the mantle. The crust and the top of the upper mantle are known as the lithosphere, which consists of tectonic plates. These plates are continuously created at the oceanic ridges (Figure 2), where hot material from the mantle is added. This material is cooled down while driven away to the subduction zones, where it is eventually consumed. Both the thickness and the density of the lithosphere increase as moving away from the oceanic ridge, leading to the progressive sinking of the sea floor and hence increasing the ocean depth.
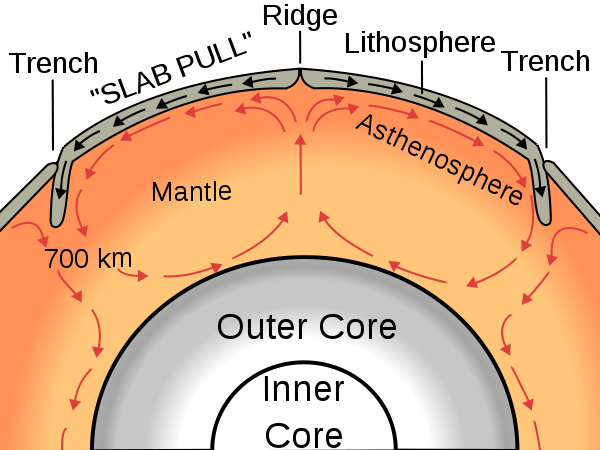
The highly viscous mantle is not steady, but it flows due to convection currents. Initially it flows outwards losing heat when it encounters a cold surface like the tectonic plates. Due to this heat loss the fluid becomes denser than the fluid rising from underneath but, since it cannot descend, it just moves to one side and continues cooling down. At some point, it becomes so dense that its weight overcomes the force of the rising fluid, and it begins to move inwards. As it descends, it warms up and the cycle starts again (Figure 2). This fluid flow pattern is characteristic of many thermal convection systems, and is known as convection cell or Bénard cell.
Let’s consider that the lithosphere is the cold upper boundary layer of the cooling mantle. In that case, its thermal structure is determined entirely by its geological age, and the sea-floor depth increases with the square root of the distance from the ridge. Then, assuming a constant plate velocity, the sea-floor depth varies with the square root of the age of the lithosphere. Traditional models based on this assumption studied the sea-floor depth along trajectories following the age gradient. However, these models present discrepancies with observed sea-floor depth at old ages (more than 70 million years ago) (see blue and green lines in Figure 3, left). These differences could be explained by an additional –yet unknown– heat supply, and for that reason, different hypotheses have been proposed. The bases of those hypotheses are unnecessarily complicated and, in my opinion, less elegant than the one reported in this article.
Rather than presenting a steady state behavior, the thermal conditions imposed on the lithosphere base change due to the temporal variations of both mantle convection and plate motion. Then, the structure of the lithosphere will adapt to the new thermal conditions, becoming thicker if the temperatures at its base are hotter or thinner if they are cooler. After several million years, the lithosphere will follow the structure of the thermal boundary layer for the new underlying mantle flow, independently of its initial state.
To prove this hypothesis, the authors analyzed profiles of the sea-floor depth of the whole Pacific plate. This plate was chosen as the best candidate for the study because it suffered a significant change in its motion due to a rearrangement of mantle convection about 50 million years ago. Since then, its conditions have remained stable, so its structure has had enough time to evolve and adapt to the new thermal conditions. Therefore, flow lines strongly differ from age trajectories. Additionally, the change in thermal conditions produced by the rearrangement of mantle flow has undoubtedly modified the structure of the lithosphere. It is estimated that the change in thickness of the lithosphere corresponding to 50 million years would be about 40 km. Finally, the velocity of the Pacific plate (the fastest) will highlight the effects of mantle convection as the driving mechanism of progressive sinking of the sea-floor.
In short, the authors propose a more simple and accurate way to reproduce the sea-floor depth by relating it to the mantle convective flow. Instead of introducing new variables in the existing model, they questioned the basis of the model itself, setting a good example of Occam’s razor.
References
- C. Adam, V. Vidal, Mantle flow drives the subsidence of oceanic plates Science 328, 83 (2010) ↩
4 comments
“Then, the structure of the lithosphere will adapt to the new thermal conditions, becoming thicker if the temperatures at its base are hotter or thinner if they are cooler.”
Why would this be? Earlier in the explanation it is said that the thickness of the lithosphere increased as it moved away from the ridge, which would imply more thickness as the temperatures at its base are cooler, not hotter… Maybe I’m missing something?
Very interesting article!
Thanks for your comments, DarkSapiens. Let’s consider a specific region of the plate. After the flow rearrangement it can either be subject to cooler or warmer temperatures, and from that moment it will move according to the new flow. If the temperatures are hotter it means that it is closer to the rising current of magma, and it still has a long way until it is consumed; time during which it will increase its width, i.e. it will be thicker than with the previous flow configuration. On the other hand, if the temperatures are cooler, it will be closer to consumption and its thickness will not increase as much as with the previous flow.
Hope this answers your question.
Ahhh that does make sense 🙂
Thanks for the answer! 😀
[…] https://mappingignorance.org/2013/03/06/mantle-convective-flow-determines-the-depth-of-the-ocean/ […]