Robotic flies manage to fly
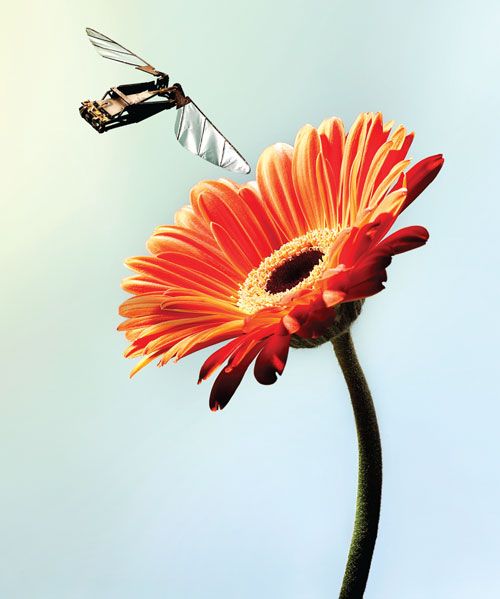
Some years ago, with a sudden and drastic reduction on the number of honeybees, scientists realized that if these insects disappeared it would be the end of agriculture as we understand it, as they are the main drivers of agricultural pollination in the US 1. But would we be able to replace these pollinators with artificial bees? Building an electro-mechanical hive has many challenges, the most important ones being the collective behaviour of the hive and the construction of small scale robots.
Biomimicry is a quite rewarding technique. Mimicking interesting characteristics of processes happening in nature allows both the achievement of new or improved techniques and a very good visibility in the media. Nothing says “look what science does!” better than a new robot design that behaves like an animal. In a recent paper in Science 2, the research group of Harvard Microrobotics Laboratory demonstrate the capabilities of a tiny flying robot resembling a common fly (see video here).
As easy as it seems, it is not trivial to miniaturize this kind of robot. The scale is so small that its weight would be around 1/100th of the smallest autonomous flying device. Autonomous means that it needs to work by itself, with no human interaction; this must include a sensory or visual system, a control system, power and the actuators that will allow the actual flight. Nowadays this seems almost impossible, but several advances are being made in all these fields that will someday allow extremely light autonomous drones. In the current case, the robotic fly only implements one of these lightweight solutions, the one regarding the actuators. Also, manufacturing the structure that will support the robot is not trivial, as mesoscale manufacturing (sizes between microscopic and conventional macroscale manufacturing) is unexplored territory.
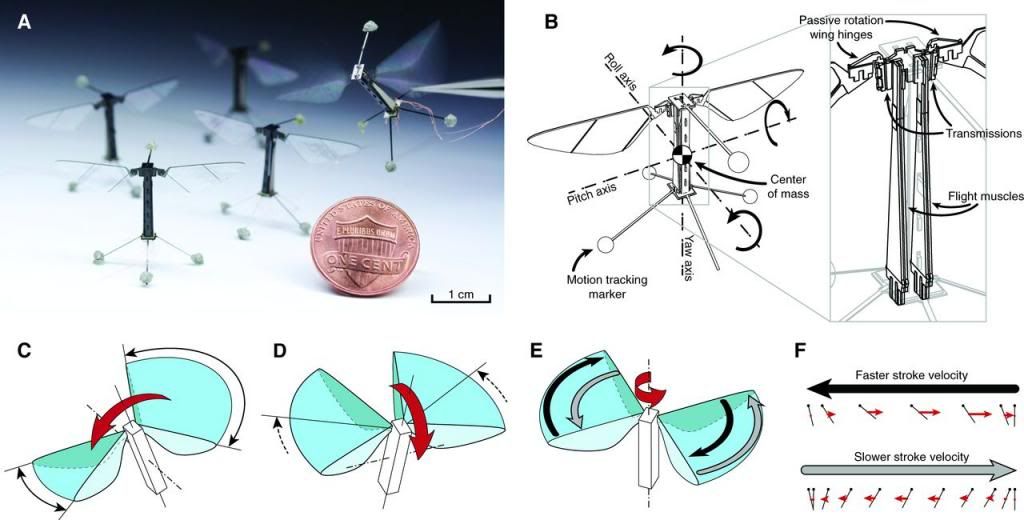
As it can be seen in Figure 1, the robot includes a supporting structure, two wings, one actuator for each wing, transmission components between the actuators and the wings, and four motion tracker markers. The power and control signals are supplied by the means of a cable attached to the base of the structure. The wing span is about 3 cm long and it weighs 80 milligrams. The use of two independent actuators with variable and controllable speed allows high manoeuvrability, enabling rotation around three orthogonal axes, meaning that it can theoretically perform any flying or hovering manoeuvre.
In order to create the different pieces of the robot, a novel technique has been developed for mesoscale manufacturing. Smart Composite Structures (SCM) consists on creating composite materials by adhering different micron-size layers of laser-micromachined materials. This enables sizes down to 5 microns, virtually countless material combinations and a capability for mass production. The electro-mechanical components of the fly were also manufactured with this technique. The materials were selected so that the relation between structural stiffness and weight was maximized.
One of the most important achievements of the research group is the actuator. The flapping of the wings has a very high energy cost, so it is necessary to achieve a very high power density. Rotary electromagnetic motors are too heavy for this duty, so authors had to search for a better alternative, and they found it in piezoelectric induced strain materials. These components generate a strain in the material when applying a current to them, kind of a mechanical muscle. They managed, using the SMC manufacturing technique and piezoelectric ceramics, to create bidirectional piezoelectric muscles and optimize their energy density. When assembling the muscles and the wings, the former control the yaw angle of the latter. This angular movement by itself does not create any vertical forces, so sustentation is not possible without a small pitch angle on the wings. To generate this angle a passive elastic joint is added, which generates this pitch angle derived from the wings’ geometry and yaw angle, enabling the appearance of sustentation forces.
The stability of high frequency and small mass devices is usually extremely bad, so they need an electronic controller that will enable them to follow the correct path. In order to control their position, speed and acceleration they need sensors that tell them their actual position, speed and acceleration. And as we mentioned before, the sensors are not included on-board. In this case control is achieved by filming the drone with several cameras in a limited enclosure that will capture its overall motion (Figure 2). Then, control signals are sent to the drone via a small wire that, according to the authors, will not affect the overall dynamics of the robotic fly. This cable both controls and powers the mechanical muscles that make wings move.
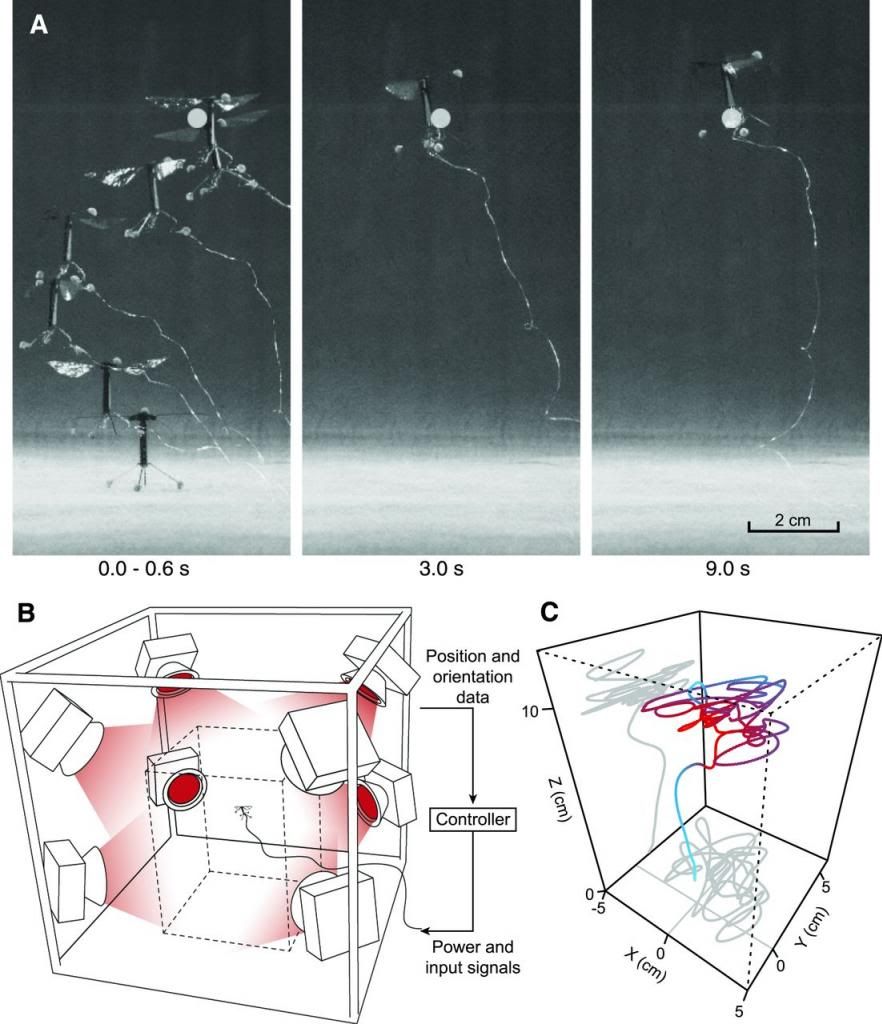
With their independent wing and variable speed control strategies they have demonstrated that the artificial fly can sustain a stable hovering around a fixed point, with errors of about one body length in position. They have also managed to perform lateral manoeuvres with quite a good fit, considering their instability level. And this is the second great achievement: they managed this with only two degrees of freedom in the robot. Two simple one-degree wing flapping mechanism is capable of performing all the possible hovering manoeuvres with the right control strategy.
The possible applications of mesoscale drones are as many as you can imagine. Apart from the artificial pollination, rescue search parties or risk assessment of dangerous places – a collapsed mine or a building after an earthquake – seem the next evident application. Having swarms of autonomous small flying robots looks quite futuristic, and in my opinion it will still be quite futuristic for a long time despite the efforts of different research groups to get us there as soon as possible. It is quite hard to include power, sensors and controllers in an 80-milligram and three centimetre robot.
References
- ‘Flight of the Robobees’ Scientific American (March 2013), 308, 60-65 DOI:10.1038/scientificamerican0313-60 ↩
- ‘Controlled Flight of a Biologically Inspired, Insect-Scale Robot’ Kevin Y. Ma, Pakpong Chirarattananon, Sawyer B. Fuller, and Robert J. Wood, Science, 3 May 2013: 340 (6132), 603-607. DOI:10.1126/science.1231806 ↩
1 comment
[…] Robotic flies manage to fly […]