Quantum mechanics in biological systems (I): Introduction
Quantum mechanics, a term continously repeated during the last twenty years, related with everything, only deeply known and understood by a few, is therefore covered in a mystic and magic halo. Halo profited by some to trick people using any sort of elfish terms containing “quantum” as a key word. To rid out of such a fraud, the best way is to know what quantum mechanics is about, delimiting its real extent. Afterwards, with more curiosity and to better understand its implications, some examples of direct and compressible effects within the living world could clarify the abstractions or, at least, place the concept in its true context, far from any hoax.
In contraposition to classical physics, which explains the macroscopic world and the forces that govern it before our eyes, quantum mechanics explains the behaviour of matter and energy at atomic and subatomic level. It provides a mathematical description of the world at picometer (pm= 1×10-12m) scale, often counterintuitive to our macroscopic point of view. The wave-particle duality, the uncertainty principle and the discontinuity of physical quantities forming packets called quantum (hence its name) are abstract concepts which describe the complicated rules of the subatomic game.
Obviously, if quantum mechanics defines the basic behavior of matter and energy, it will indefectibly govern biological systems. Nevertheless, evidence of its direct operation in living systems has been slow to show up. The reason of that is the quantum decoherence, another complex term which in simple words says that a physical system stops showing quantum effects and becomes understandable under the classical physics framework because the quantum behaviour vanishes averaged by the noise of uncontrolled interactions in a highly complex environment. To make it easier, lest’s imagine Schrödinger´s cat (inside the box) whose life hangs on a single atom disintegration. In a quantum mechanic scale, the cat is alive and dead at the same time, because that single disintegration happens or not at the same time. In our scale the cat is either alive or dead. So, the quantum decoherence are all those phenomena impairing us to see an alive-dead cat. Those phenomena are noise, disturbances or interactions coming from the fact that this single atom is not alone there. The cat moves, the air changes, the molecules hit each other, you open the box… any event could affect the integrity of a single atom triggering its disintegration, the choice: either dead or alive.
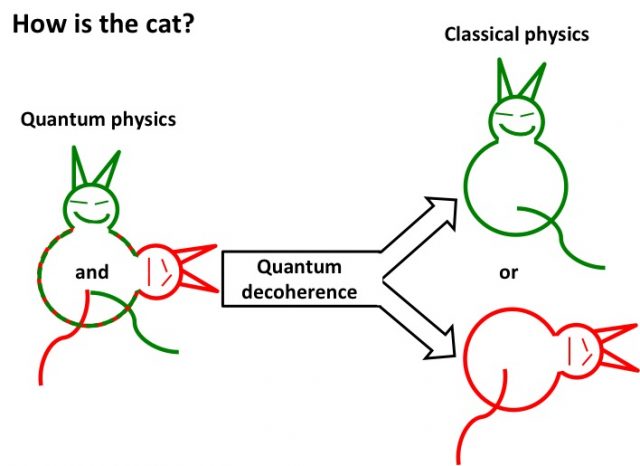
After centuries studying the biological systems, lately even with increasingly powerful microscopes, no clue emerged about quantum behaviour within the living world. The biological realm is characterised by complex environments even at molecular scale. Therefore, quantum decoherence seemed to hide the weak quantum effects. But in the last two or three decades, some technologies rose, more and more accurate in the measurement and robust in the control, making possible the identification of quantum effects in biology 1. Beyond those quantum effects observable in the living matter just because it is matter, there are several examples of functional necessity. Here the quantum effects are essential to get the biological system working. Hence, they are susceptible for natural selection and evolution. Gecko´s adhesion, magnetoreception in birds, human odour recognising and light harvesting dynamics in photosynthetic systems are examples being discussed in this series talking about quantum mechanics in biological systems. Hoping that this emerging science field called quantum biology helps to show the true scope of quantum mechanics in living systems.
References
- Sarovar, M. Quantum effects in biological systems. 75610D–75610D (2010). doi:10.1117/12.841015 ↩
4 comments
Could you send me the links of the references? It seems they doesn´t work
Dear uleon,
Now they should work.
Cheers
[…] Quantum physics […]
[…] Quantum mechanics in biological systems (I): Introduction […]