Precise cell division and cognition in modern humans
Cell division, or mitosis, is a breathtaking choreography that showcases the grandeur of life. The primary objective of this process is to meticulously separate the two copies of the cell genome, presented in the form of chromatin, and allocate them between two daughter cells. Consequently, it holds paramount significance for the reproduction of unicellular organisms and plays a crucial role in the growth, differentiation, and proper functioning of tissues in multicellular organisms. Furthermore, it has been suggested the its precision may correlate with the expanded count of neocortical neurons and the development of cognitive abilities that characterize modern humans.
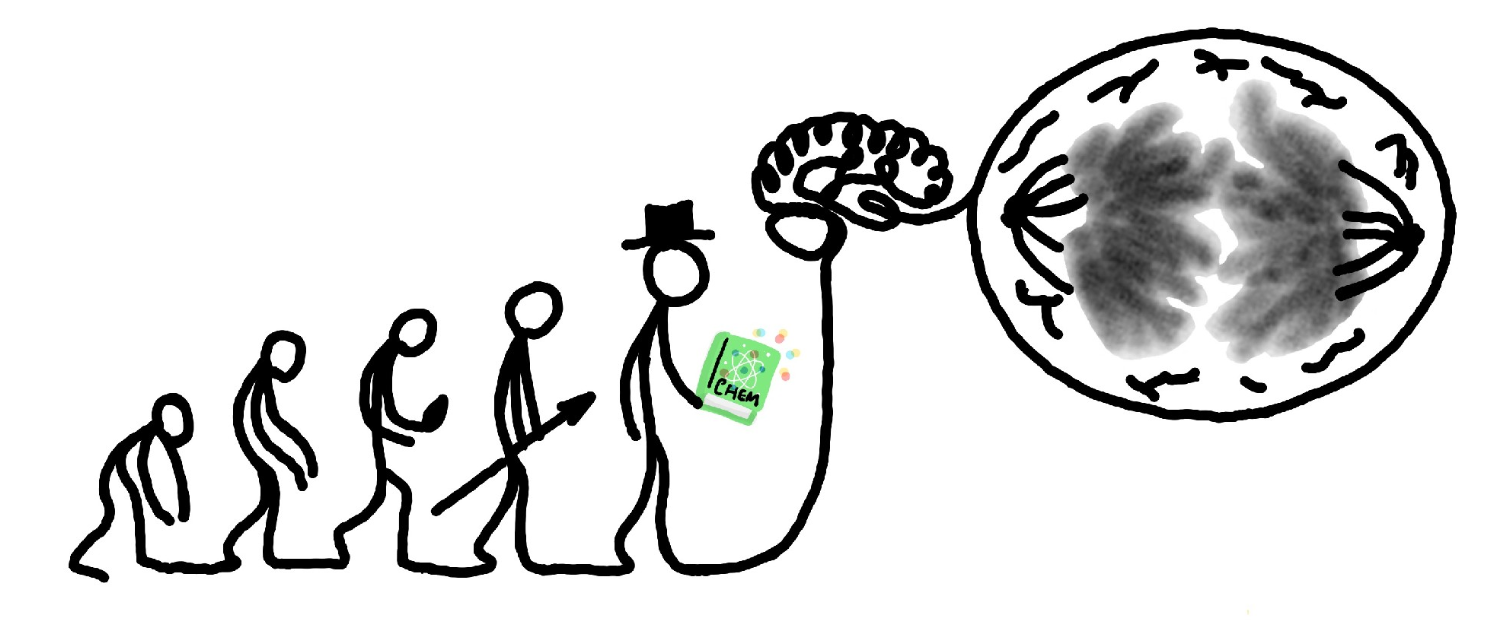
Mitosis is arguably the most significant restructuring event a cell undergoes throughout its lifespan. This phase of a cell’s life has traditionally been divided into sub phases based on the characteristics of the chromatin mass and other cytological hallmarks. In this manner, we refer to ‘prophase’ as the period when the nuclear envelope breaks down, and the chromatin condenses to form the characteristic rod-shaped chromosomes. The ‘prometaphase’ is the timeframe during which the individualized, spread chromosomes commence their dance and organize themselves on the metaphase plate. The ‘metaphase’ continues until all the chromosomes are perfectly aligned and correctly captured by the spindle apparatus. The mitotic spindle is a remarkable molecular micromachinery capable of positioning and pulling apart the two chromatids of each chromosome. Then, just when everything appears in order, the onset of ‘anaphase’ occurs. During anaphase, the spindle forces segregate each chromatid to opposite cell poles. In the subsequent ‘telophase’, the compact chromosomes slowly decondense while the nuclear envelope covers the chromatin mass, re-establishing the functional nucleus. Concurrently, the spindle disassembles while the bodies of the two emerging daughter cells become discernible, owing to the molecular machinery that ultimately constricts the cell membrane between the two newly formed nuclei in a process known as cytokinesis.
A plethora of molecular processes are required to orchestrate the timely dance and accurate separation of chromosomes. The mechanisms of mitotic control are diverse, relying on a multitude of proteins that modulate the molecular machinery responsible for capturing and separating chromosomes, ultimately giving rise to the daughter cells. Among these proteins are those that constitute the spindle assembly checkpoint, a network that governs how the spindle apparatus at each cell pole effectively captures chromatids during metaphase. This crucial checkpoint network delays the onset of anaphase until all chromosome-spindle attachments are correct, ensuring proper chromosome segregation. Consequently, any dysfunction or reduced activity of the proteins in this regulatory network could lead to errors, resulting in cells with incorrect genetic material (see Figure 1). This unfortunate circumstance can, in the short term, lead to cell death. Alternatively, if the cells manage to survive the erroneous mitosis, they may experience malfunctions or even become cancerous 1.
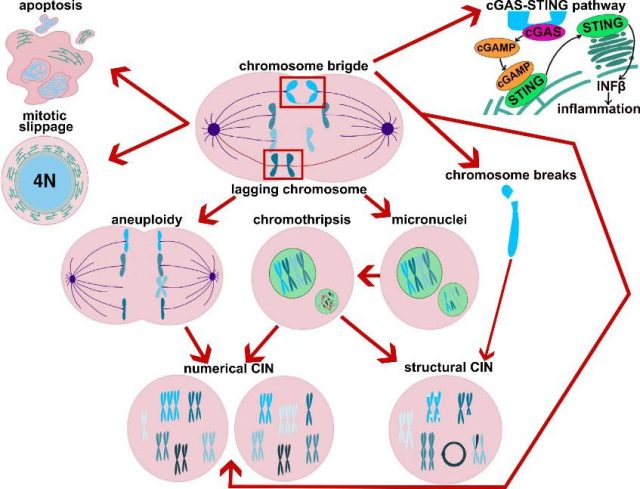
However, what does mitosis have to do with the drastic increase in size of the modern human cerebral neocortex and the associated cognitive abilities? In a 2016 study 2, researchers could observe live mitosis in specific types of stem neocortical cells across several species using organoids (see note 1) of proliferating apical progenitors. They discovered that human apical progenitors spend approximately 50% more time in metaphase compared to those of chimpanzees and orangutans. Since longer metaphases provide more time to correct potentially defective chromosome alignments, this suggests a more robust chromatin segregation process. Interestingly, there were no extended metaphases when comparing other types of stem cells from non-neural germ layers across species.
Given these findings, it was tempting to speculate that a more efficient and less error-prone mitosis could lead to a higher rate of neuron production and enhanced fitness of these neurons. As a result, Mora-Bermudez and colleagues 3 conducted a study characterizing the function of three proteins—KIF18a, KNL1, and SPAG5—across species. These proteins play known roles in mitosis and spindle checkpoint function, are highly expressed in the developing neocortex, and share amino acid sequences common to all modern humans, but different from those shared by Neanderthals, other archaic humans, and chimpanzees.
The research demonstrated that the expression of the modern-human version of the proteins KIF18a and KNL1 led to longer metaphases and fewer errors in chromosome segregation compared to the Neanderthal-like forms of these same proteins (see note 2). Furthermore, they showed that this could be attributed to the existence of a much more robust spindle assembly checkpoint, as indicated by the functional assessment of several spindle molecular markers. However, the expression of modern human proteins in mice did not affect the neocortical development, and the overall morphology, size, and shape of the neocortex in adult humanized mice remained unchanged.
All of this may have significant evolutionary implications in the neocortical development. In the early stages of cerebral development, apical progenitor cells undergo symmetric divisions to increase their numbers. In this context, the more robust mitosis observed in modern humans may amplify the number of these valuable stem cells, which would be less affected by cell death resulting from chromatin segregation errors. A bit later in neocortical development, apical progenitor cells undergo asymmetric divisions to generate the various cellular components of the neocortex (see Figure 2). In this scenario, the more accurate chromosome segregation in modern human progenitor cells may confer superior functionality to the entire cortical column derived from a single apical progenitor. Therefore, this work suggests that the amino acid substitutions in some mitotic regulators that occurred during evolution between Neanderthals and modern humans could contribute to explaining the development of cognitive abilities characteristic of modern humans.
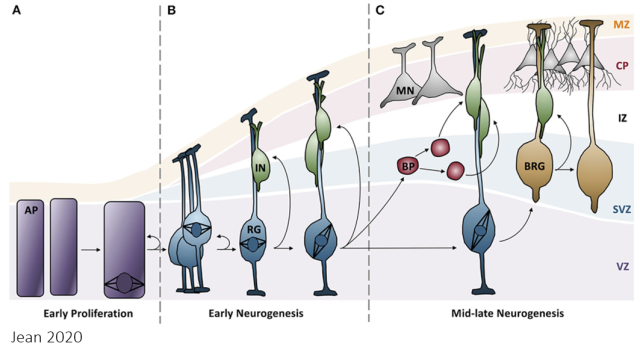
Numerous attempts have been made to elucidate the evolution of the human brain from various perspectives and at different scales. In a previous discussion on Mapping Ignorance, we delved into the “expensive brain hypothesis” (see https://mappingignorance.org/2015/02/13/muscle-vs-brain-perhaps-matter-energy-expenditure/), which endeavors to clarify why and how our brains evolved to require several times more energy than those of other mammals and primates of similar size. Given the above recent observations, it is tempting to speculate that the high mitotic fidelity in neocortical stem cells may be the driving force behind our abundance of neurons and the significant energy expenditure, which could be associated with heightened cellular fitness.
In this line of thinking, mitotic fidelity could be linked to the more advanced cognitive abilities exhibited by modern humans in comparison to ancestral humans and apes. However, it remains unclear whether the expression of archaic or modern forms of these two mitotic regulatory proteins ultimately has a discernible impact on neocortical development and the overall characteristics of the neocortex in adult hominids. Neanderthals, for instance, possessed markedly larger brains and cognitive abilities well beyond those of chimpanzees, despite both species sharing the same sequence of these three proteins.
All of this implies that the number of cells, their development and functioning in the neocortex may not be exclusively tied to the precision of the mitotic event. Therefore, the research presented today should be approached with caution. As the authors suggest, further research is needed to determine whether these molecular changes hold significance for our cognitive traits. It is highly likely that the evolutionary alterations in these two proteins, on their own, did not exert a major impact. Rather, when combined with other molecular changes of similar magnitude, they contributed to the emergence of a phenomenon that transcended genome divergence.
Notes:
Note 1: ”organoids are simple tissue-engineered cell-based in vitro models that recapitulate many aspects of the complex structure and function of the corresponding in vivo tissue” 4
Note 2: by mean of different cellular models, cerebral organoids and live-tissue imaging organotypic slice cultures of embryonic mice neocortex.
References
- Moreno-Andrés, D., Holl, K. & Antonin, W. The second half of mitosis and its implications in cancer biology. Seminars in Cancer Biology 88, 1-17, doi: 10.1016/j.semcancer.2022.11.013 (2023). ↩
- Mora-Bermudez, F. et al. Differences and similarities between human and chimpanzee neural progenitors during cerebral cortex development. Elife 5, doi: 10.7554/eLife.18683 (2016). ↩
- Mora-Bermúdez, F. et al. Longer metaphase and fewer chromosome segregation errors in modern human than Neanderthal brain development. Sci Adv 8, eabn7702, doi:10.1126/sciadv.abn7702 (2022). ↩
- Zhao, Z. et al. Organoids. Nature Reviews Methods Primers 2, 94, doi:10.1038/s43586-022-00174-y (2022). ↩