Inside a cell, no one can hear you turn into an infective zygote
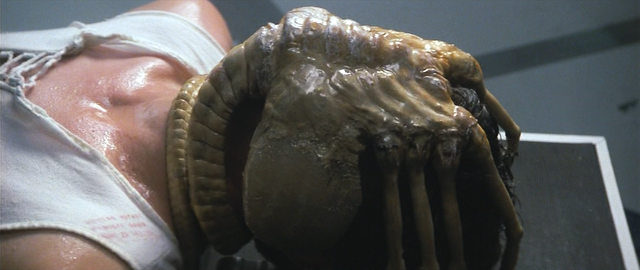
It is common to think that sci-fi writers are extremely creative and smart when they depict alien creatures with weird (and usually disgusting) ways of reproducing, generally using for this purpose the bodies of incautious and often naïve human characters. And they truly are that creative and imaginative, I’m not going to take that credit from them; but the fact is that if one pays close attention to the lives of the parasitic organisms that cause diseases among us, then those artificial creatures will not seem as menacing and terrifying anymore. A good example is the tiny, unicellular parasite Plasmodium, causative agent of the devastating disease that we call malaria. Its reproductive life cycle, which involves living in environments as different as the bloodstream of a person or the guts of a mosquito, requires extraordinary transformations of its physiology. Since we know that those transformations are quite easily directed by the control of only a small number of genes (those molecular switches we talked about here), it is crucial to define what those genes are activating or deactivating, in order to pursue the final solution for the pathologies associated with their infection. Today’s paper focuses on one of these switches, dissecting the consequences of its disruption on the physiology and infective capacities of the parasite. It is a very nice example of the benefits of multidisciplinary science and the goals achieved thanks to the implementation of bioinformatic, biochemical and genetic engineering techniques.
The work by Patzewitz and collaborators 1 starts with a bioinformatics analysis of a particular group of proteins which very commonly act as molecular switches: the protein phosphatases. Tracking track back the information encoded in the genes that correspond to very similar proteins (this is called phylogenetic analysis) is a very nice start to investigate unknown functions. In today’s example, researchers centered their attention in a pair of relatively odd phosphatases from all the different ones included in the Plasmodium genome. They are similar to other phosphatases present in humans, but different enough as to be considered a separate group. It is easy to understand the importance of confirming that a certain protein exists only in a parasite organism, but not in the ones it infects, from a medical point of view. So, making the long story short, the main characters of this tale are proteins with a bacterial origin (the Shewanella genus) which are not present in animals: they are called SHLP1 and SHLP2 (Shewanella-like Phosphatase 1 and 2). Identified from a few years ago, nobody knew about their molecular functions and their relevance for the parasite’s life. We will now follow the work by Patzewitz and collaborators, which involves SHLP1, and uncovers really interesting facts.

Methodologically, working with such a shape-changing parasite, one of the first data to collect is the precise moment in which the protein appears into place. Very briefly, let’s remember that the parasite’s life cycle includes periods inside a mosquito and inside a mammalian host; during the course of this incredible voyage the unicellular body changes substantially and acquires different properties: male and female sexual gametes unite to form a zygote, which then turns into a mobile ookinete, then an oocyst, and finally divides asexually into sporozoites which are the ones transfected into the animal after mosquito biting. SHLP1 was present in all stages but was more abundant in the male gamete and the oocyst. Good news, since this could mean an important role in the Plasmodium life cycle.
The next approach was knocking down the expression of the protein (i. e. generating a genetically altered parasite, in which SHLP1 is eliminated: a KO parasite) and waiting to see the effects on the parasite’s normal way of life. For starters, it did not die, so whatever the protein was doing was not critical for parasite survival. However, following the progression of this KO parasites revealed that the generation of ookinetes was severely reduced in this conditions. To further track this clue, the researchers let the modified parasites infect alive mosquitoes and observed if the ookinetes could transform into their natural next developing step, the oocyst: they got the surprise that no oocyst could be formed starting from SHLP1 KO parasites. After some more experiments, the final conclusion was that almost all of the parasite’s life forms were able to be reached in SHLP1-lacking parasites, but the concrete step from ookinete to oocyst was drastically impeded. In order to find an explanation for this interesting effect, they made a jump from the molecular scale and studied the parasite using microscopy techniques. This method revealed significant changes in a particular structure of the organism called micronemes. These are small membranous structures filled with proteins with a secretory role, like balloons that are expelled outside the parasite in order to liberate a series of proteins which facilitate, somehow, the invasive capacities of the Plasmodium inside its host. As shown in the impressive images below, there is an evident reduction in the number of micronemes in the parasites lacking SHLP1, and the few that are present appear located in a wrong position. Again, this gives the impression that the mutant form of the parasite deficient in the phosphatase SHLP1 will have serious problems to perform the attack and posterior invasion of the host. A molecular confirmation of this observation is that the genes responsible for construction of several proteins with this aggressive role, are severely downregulated in the mutants.
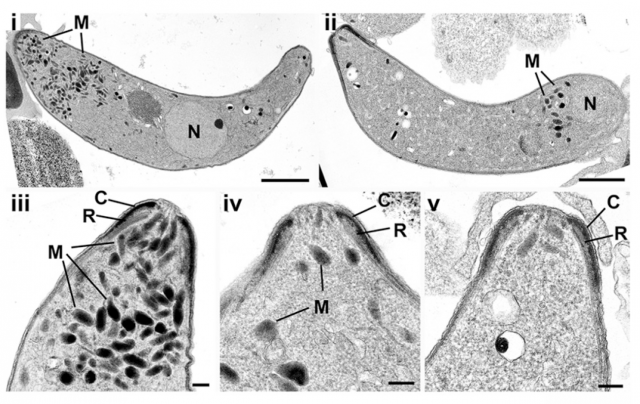
Up to this point, we can conclude that the presence of the phosphatase SHLP1 is critical for Plasmodium to become a successful invader of other organism’s cells. The authors also proved that this protein is an active phosphatase, which suggest that whatever it does inside the parasite is done by means of this enzymatic activity. However, what he target of this activity is, remains a mystery. But fortunately, mysteries in science are a matter of time. For instance, the “sister” protein SHLP2 has been recently shown to act as an active phosphatase, accumulated in granules similar to the micronemes, and involved in the dephosphorylation of a protein present in the host’s blood red cells 2. Studying the similarities and differences of both proteins may help to direct the efforts in finding out the actual target of SHLP1. But here are the good news: we usually don’t need to get as specific as that. The present work is a good example of how nowadays we can trace the participation of a protein in certain cellular processes and discover the effect of its elimination. If we take into account that it is sufficiently different to our own proteins, designing a chemical product that inhibits its activity or affects its biochemical properties can constitute a promising strategy to avoid the spreading of the parasite from the mosquito to human beings. It may seem a difficult task, and indeed it is; but we have already several examples in which molecular strategies in medicine are becoming a turning point in the fight against many disease.
In the end, even though the terrifying alien creatures from the aforementioned films are hard to kill, they can be hit, shot or burnt down But we are fighting microscopic intruders which travel hidden in small invertebrates: certainly the molecular approaches are our only hope.
References
- Patzewitz E.M., Guttery D., Poulin B., Ramakrishnan C., Ferguson D.P., Wall R., Brady D., Holder A., Szöőr B. & Tewari R. & (2013). An Ancient Protein Phosphatase, SHLP1, Is Critical to Microneme Development in Plasmodium Ookinetes and Parasite Transmission, Cell Reports, 3 (3) 622-629. DOI: 10.1016/j.celrep.2013.01.032 ↩
- Fernandez-Pol S., Slouka Z., Bhattacharjee S., Fedotova Y., Freed S., An X., Holder A.A., Campanella E., Low P.S. & Mohandas N. & (2013). A Bacterial Phosphatase-Like Enzyme of the Malaria Parasite Plasmodium falciparum Possesses Tyrosine Phosphatase Activity and Is Implicated in the Regulation of Band 3 Dynamics during Parasite Invasion, Eukaryotic Cell, 12 (9) 1179-1191. DOI: 10.1128/EC.00027-13 ↩