Melodies of proteins
The way in which nature manages to create functional diversity in matter is probably the wisest guide for biomaterials engineers, who are always looking for new creative processes to come up with new materials. The conventional way of designing complex materials with new functionalities is explained by the so-called top-down approach, in which a new function is achieved by adding new raw materials to the previous formulation. In other words, complexity is achieved by means of addition. In contrast, the bottom-up approach tries to mimic nature’s ability to create functionality by using a few number of simple building blocks. From this point of view, complexity is achieved by a proper assembly of scales, from nano to macro-scale, in a hierarchical structure.
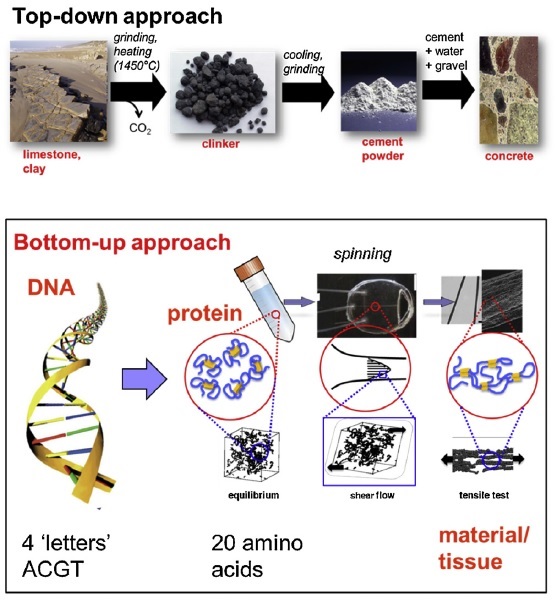
Let’s have a look at proteins engineering. Proteins are large biological molecules performing a wide range of functions in living systems and consisting of different sequences of amino acids. There are just 20 standard amino acids acting as building blocks for proteins, and it is through a complex structural organization that a vast range of biomaterials are obtained from this limited library of 20 fundamental compounds. The same paradigm can be found in the field of music: just a few notes, tones and rhythms lead to an infinite variety of melodies in which the distinguishing factor is the way in which these pieces are hierarchically structured during the creative process.
This idea of achieving complexity through complex organization of a limited number of building blocks has not been overlooked by biomaterials designers, as it seems a sustainable and inexpensive way of creating new nanomaterials with new functionalities. In a recently published article1, an interdisciplinary group of researchers, comprising biomaterials engineers, mathematicians and musicians from Berkeley and the MIT, have put together their particular backgrounds to obtain new bioinspired materials translating the hierarchical structure of different physical systems into a mathematical model through category theory. This categorization of physical systems reveals the rules governing the construction of the system leading to an abstraction which can be later applied in the design of new systems in different domains.
The authors propose a thrilling experiment: is it possible to encode the hierarchical structure of silk and then translate it into a musical piece? And if so, how does this music sound?
Silk is a natural protein fiber made up of two main building blocks arranged on a repetitive basis, the first one being the hydrophobic domain (domain A from now on), which leads to stiff and strong regions, and the second one being the hydrophilic domain (domain B), more disorganized, softer and extensible. Both of them are joined by hydrogen bonds (H). The study starts with the development of two different synthetic silk-inspired materials in which the only difference is the sequence of their protein building blocks. In the first sequence the hydrophobic domain (A) prevails over the hydrophilic one (B), the main repetitive unit being HA3B. On the other hand, in the second sequence prevails the hydrophilic domain, with the sequence HAB3. As might be expected, an increasing amount of A will lead to stiffer and stronger proteins while increasing B will result in more deformable proteins.
Taking these units as a starting point, and following the bottom-up philosophy, the next challenge would be to connect the nanoscale with the macroscale creating functional diversity in this way up through the scales of matter. Here again, the authors have paid attention to how nature works by mimicking the insects spinning of silk-proteins into fibers. By means of an experimental microfluidic flow approach they tried to follow to the next scale, the fiber formation.
The results were quite different in both materials. While the HAB3 sequence proved able to form fibers, the sequence HA3B, stiffer and stronger, didn’t. Using Dissipative Particle Dynamics modeling (a stochastic technique for simulating the behaviour of fluids) the authors have been able to explain this result. It seems that in the HA3B unit the hydrophobic interactions are so strong that they lead to unconnected globular structures unable to scale up their properties to larger length scales. However, the HAB3 unit, abounding in flexible interactions, is able to scale up its properties through fiber formation, as it can be seen in figure 2.

Once a hierarchical assembling has been achieved and the emerging materials’ properties have been understood by means of testing and simulation, the next step will be the categorization of this new system. Category theory is a strong mathematical tool capable of codifying all the underlying interactions of the physical system under study in order to achieve an abstraction which describes structure-properties relationships, then allowing to envisage how function emerges from hierarchical systems.
This category theory representation of the system can be later applied to different systems in other domains, and that is what the authors have done by translating the hierarchical structure of these synthetic silk materials in their fiber formation into a musical piece. While in the first domain we had amino acids as building blocks, in the new domain our fundamental pieces are notes. While amino acids gain complexity through proteins formation creating their own networks, the music building blocks will acquire the same complexity by creating chords and melodies.

According to the set of rules governing the behaviour of the silk-inspired materials the authors have constructed different melodies. They have divided the resulting melodies in “non-fiber forming” and “fiber-forming” melodies. Interestingly, the music derived from non-fiber forming materials has been described as aggressive and sharp; on the contrary, music derived from fiber forming materials was soft and lyrical, connecting with the idea of an integrated structure.

This transition from materials science to music and vice versa is a stimulating example of the potentiality underlying this concept of understanding hierarchical systems through category theory. Once we have gathered all the complex information of a physical system, we will be able to translate its hierarchical structure into a different domain, thus allowing, as it is the case in the present study, the design of new nanomaterials with new functionalities. Do you dare to imagine the properties of the matter emerging from Beethoven’s work?
References
- Wong J. Y., McDonald J., Taylor-Pinney M., Spivak D. I., Kaplan D. L., Buehler M. J., Materials by design: Merging proteins and music, Nano Today (2012) 7, 488-495. ↩