Craving for minerals: the delicate balance of the energy transition
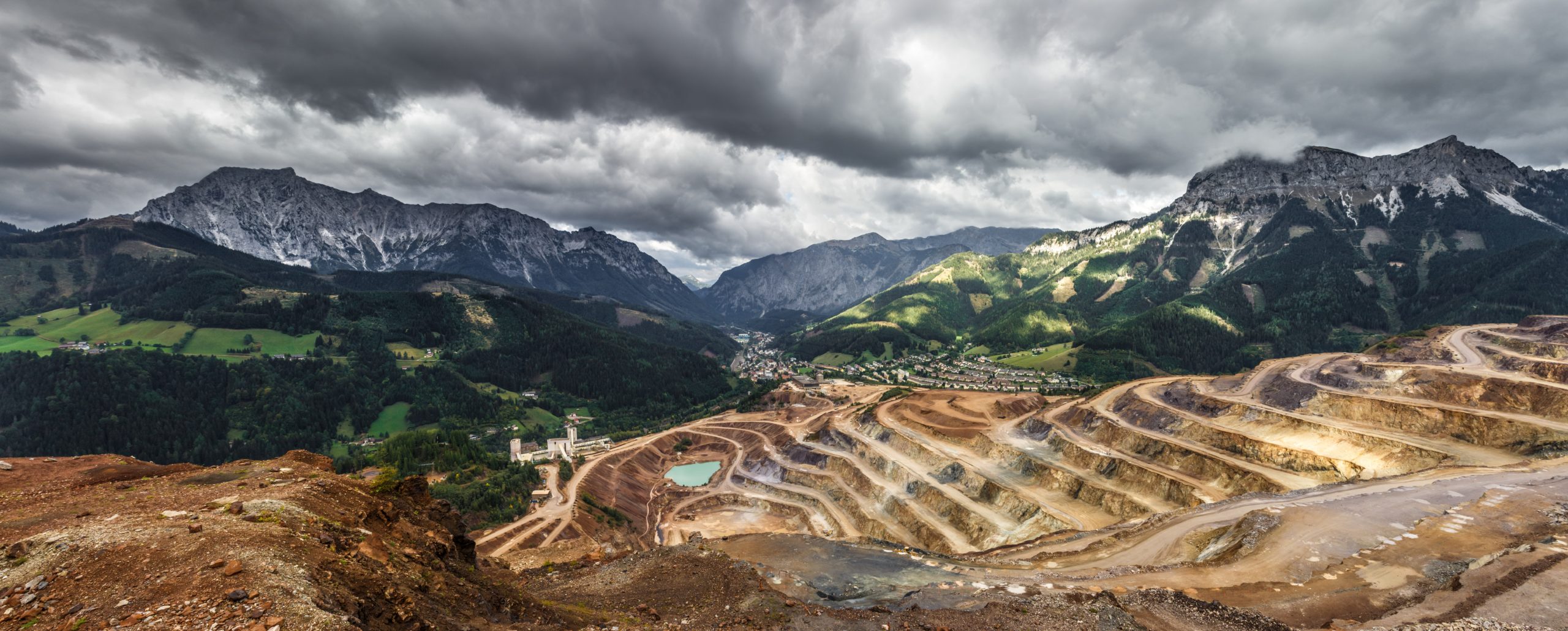
Even if we firmly go down the path of the low-carbon economy, the energy transition to renewables is only just beginning. We live now in a world that is still very much fossil fuel-based and the landscape of a fully renewable-powered future has not yet been shaped (and we might have to wait a few more decades to see it). At the moment, there is a great deal of uncertainty about which technologies will prevail and become dominant in the market and which ones will be discarded and rapidly forgotten. What we really know for sure is that most of the technologies and subtechnologies that are presently being developed are highly dependent on the availability of a variety of minerals, not a minor issue both from the environmental and socio-political perspectives.
It is a fact that the energy transition will be mineral-intensive, and thus it will come at a cost in terms of consumption of natural resources. According to a recent report1 from the World Bank Group, the production of minerals such as graphite, lithium and cobalt could increase up to 500% by 2050 just to meet the demand for clean energy technologies and even if recycling rates increase largely they will be far from meeting the demand for minerals that are critical to produce these technologies. In addition, the already mentioned uncertainty about which precise mix of technologies will succeed in the market makes it more difficult to assess the natural resources at risk.
But let’s have a quick look at the main technologies at stake and their dependence on specific minerals. It goes without saying that solar photovoltaic and wind are the fastest growing and most widely deployed renewable energies worldwide, having achieved electricity generation prices competitive with those of fossil fuel generation. Regarding solar PV, it is expected that crystalline silicon cells remain dominant in the market, although other subtechnologies such as copper-indium-gallium-selenide (CIGS) cells could increase their market share due to some interesting features, such as flexibility and reduced thickness. At any rate, prospects are that aluminium will account for 88% of solar PV components by 2050, followed by 11% of copper and a mix of other minerals such as silver, lead or indium. And even if aluminium has the highest production levels compared with other less common minerals used in renewables, this element will become vital not only for solar PV but also for other related technologies such as energy storage. Aluminium becoming a strategic material for the energy transition can be deduced from the recent incorporation of bauxite, the main mineral source of aluminium, to the 2020 EU list of critical raw materials2.
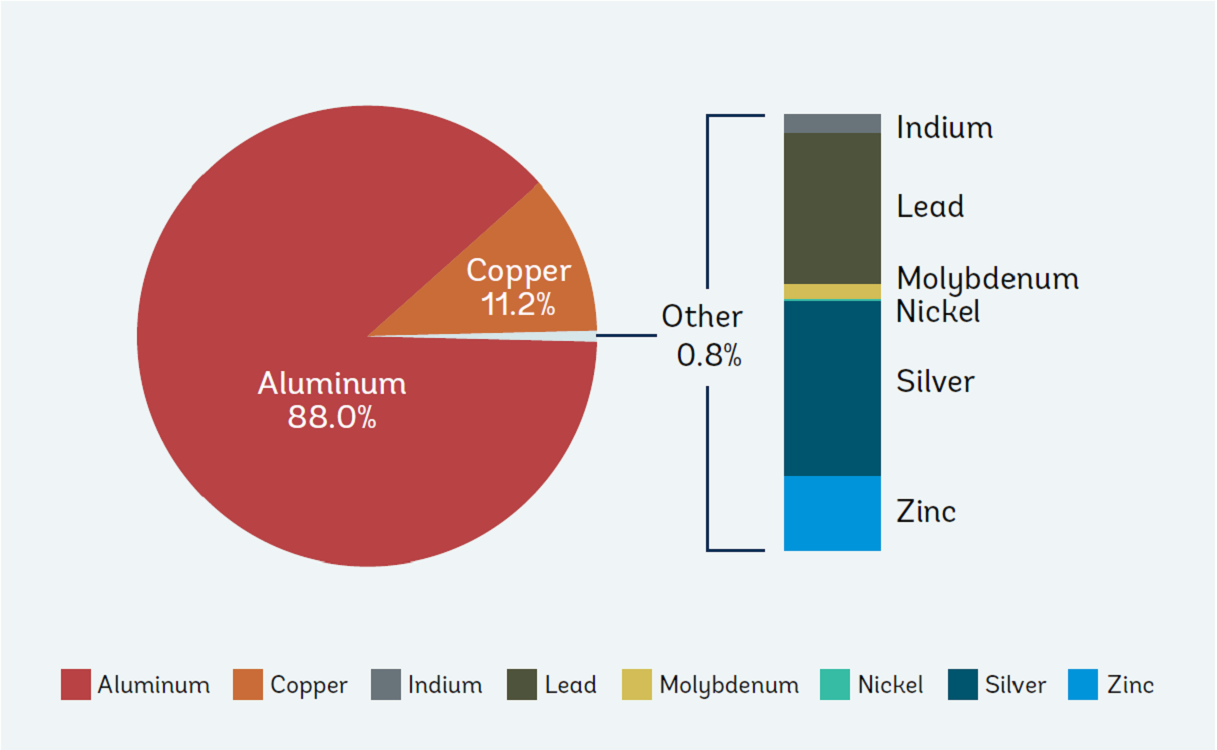
Wind energy has developed two main solutions based on different turbines’ location, onshore and offshore. Both solutions carry their corresponding subtechnologies: onshore mostly uses geared turbines, with iron and copper as their main components, while offshore uses direct-drive turbines, where the frequent use of permanent magnets involves small quantities of rare earth minerals such as neodymium and dysprosium. The changing share of onshore/offshore subtechnologies will have an impact on the demand of these rare earth minerals that, if not scarce, have extraction and production processes extraordinarily damaging to the environment.
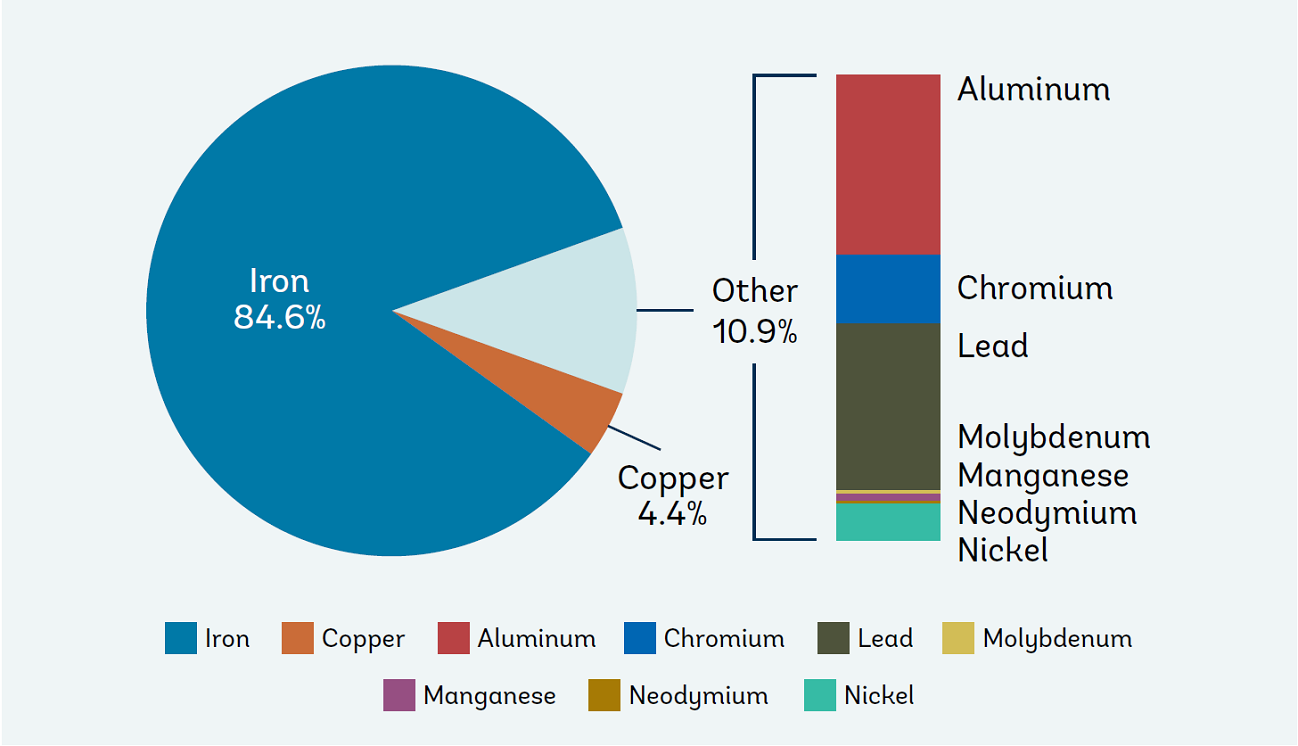
The third relevant technology towards a low-carbon future is undoubtedly energy storage. It will be key to store the power intermittently generated by solar PV and wind farms and to later dispatch it as needed. Moreover, energy storage is already being pivotal in the development of electric vehicles. Both applications, stationary and mobile energy storage, have different desirable features and thus will result in different technological solutions (for instance, weight is key in vehicles but somehow irrelevant in stationary applications). Although energy storage subtechnologies are currently one of the most changing innovation landscape, some cross-cutting minerals used in batteries will have a central role in the near future, specifically graphite (used in the Li-ion battery anode), cobalt and nickel (part of some Li-ion battery cathodes) and lithium (present as the electrolyte in the form of lithium salts).
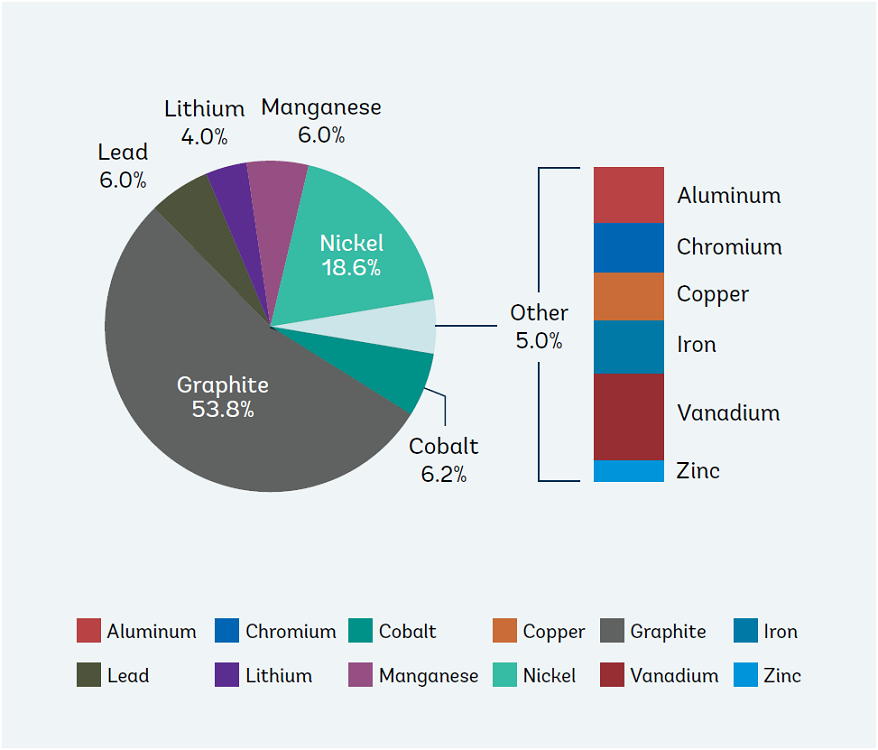
These prospects, that have been carefully assessed by global institutions such as the International Energy Agency (IEA) and the International Renewable Energy Agency (IRENA), raise the issue of mining becoming a key agent of the energy transition, a reality that it could be described, at the very least, as shocking. Extractive activities have indeed remarkable impact both on the environment and on local communities, not to mention the global instabilities caused by transnational raw materials trade. Failure to address these environmental and socio-political risks could well result in a major setback for climate action, condemning us to repeat the old patterns inherited from the fossil fuel era.
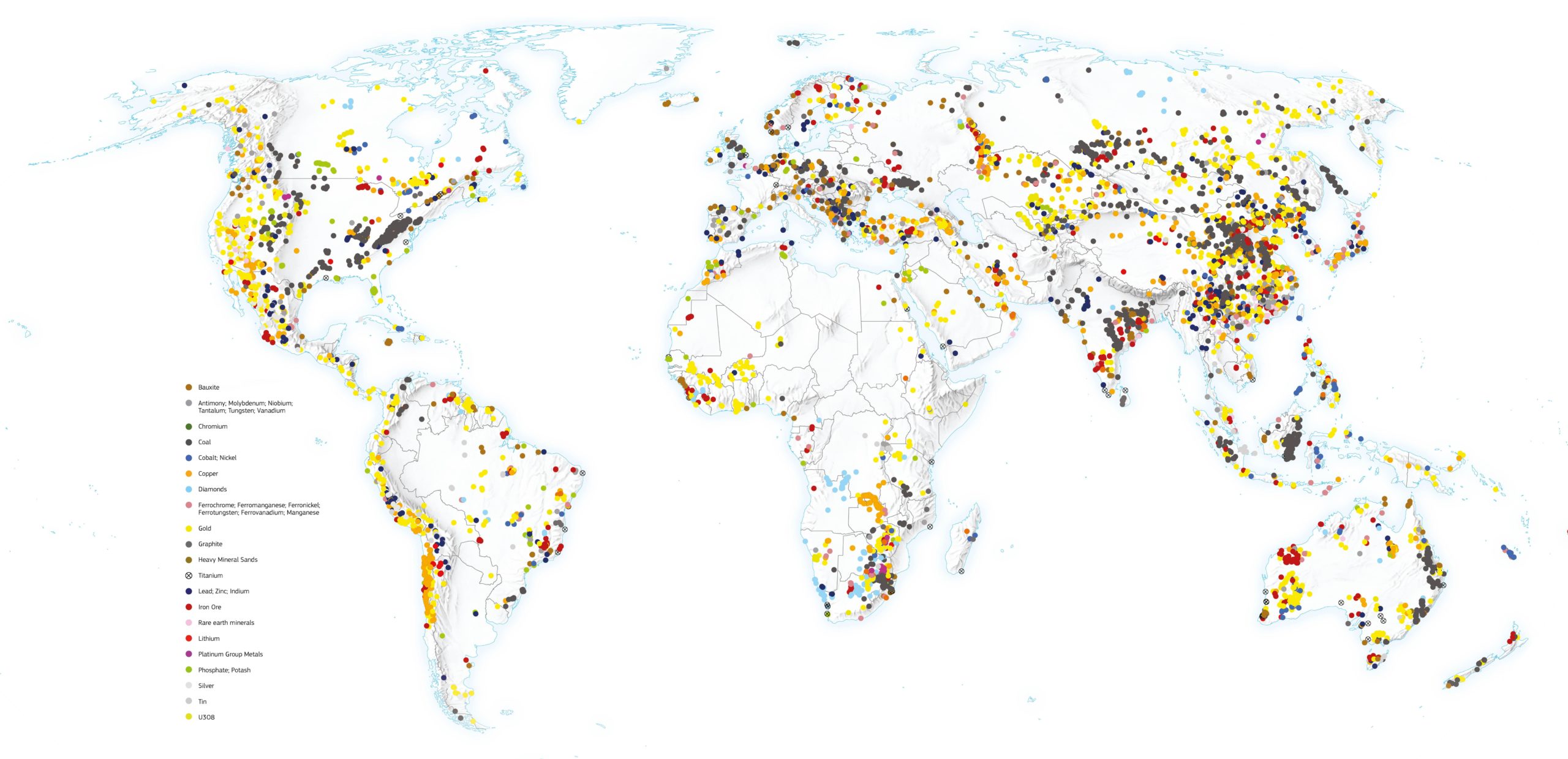
A recently published article3 raises awareness about the geopolitical consequences of the energy transition, pointing out that increased competition over a certain group of minerals, unevenly distributed around the globe, can bring new risks and tensions. Ensuring an uninterrupted supply of the materials needed for the energy transition is currently a major objective of international actors such as the European Union. We already mentioned the renewed 2020 list of critical raw materials launched last September by the European Commission, a list where supply risk is an essential parameter to assess the material ‘criticality’. In fact, some of the new incorporations to the list are closely related to high supply concentration: 64% of bauxite EU imports come from Guinea and 78% of lithium comes from Chile4, two minerals identified as pivotal for the energy transition. Not to mention China’s quasi-monopoly on rare earth elements exports such as dysprosium and neodymium.
As for the impact on the environment, we cannot lose sight of the fact that extraction of finite natural resources always comes at the expense of ecosystems and the peoples inhabiting them (that is, after all, the whole point of transitioning to renewables). Scientists from Australia and Canada, two countries closely linked to the mining sector, are currently analysing5 how mining activities specifically addressed to renewable energy production will exacerbate threats to biodiversity. They have mapped the global extent of areas potentially affected, concluding that up to 50 million km2 of Earth’s land surface are at risk of suffering the consequences of these extraction activities, 8% of them coinciding with protected areas, 7% with key biodiversity areas and 16% with remaining wilderness, ie, areas currently free from industrial-scale activities and human pressures. Among the main threats to species and ecosystems derived from mining activities are soil degradation due to acid mine drainage, deforestation and water stress and contamination6.
All in all, the results of the latest analyses suggest that achieving a large-scale deployment of renewable energy will require a delicate balance between meeting minerals demand and allowing land and biodiversity protection. Experts advise not to take anything for granted and strategically plan our future energy system, avoiding the mistakes of the past and introducing the energy transition weaknesses and contradictions into the public debate.
References:
1 Minerals for Climate Action: The Mineral Intensity of the Clean Energy Transition, World Bank Group, 2020.
3 Vakulchuk R., Overland I., Scholten D. Renewable energy and geopolitics: A review. Renew Sustain Energy Rev 122 (2020) 109547. https://doi.org/10.1016/j.rser.2019.109547
4 European Commission, Study on the EU’s list of Critical Raw Materials – Final Report (2020).
5 Sonter, L.J., Dade, M.C., Watson, J.E.M. et al. Renewable energy production will exacerbate mining threats to biodiversity. Nat Commun 11, 4174 (2020). https://doi.org/10.1038/s41467-020-17928-5
6 Cherlet, M., Hutchinson, C., Reynolds, J., Hill, J., Sommer, S., von Maltitz, G. (Eds.), World Atlas of Desertification, Publication Office of the European Union, Luxembourg, 2018.