Critical materials: The missing piece of the “green economy” puzzle
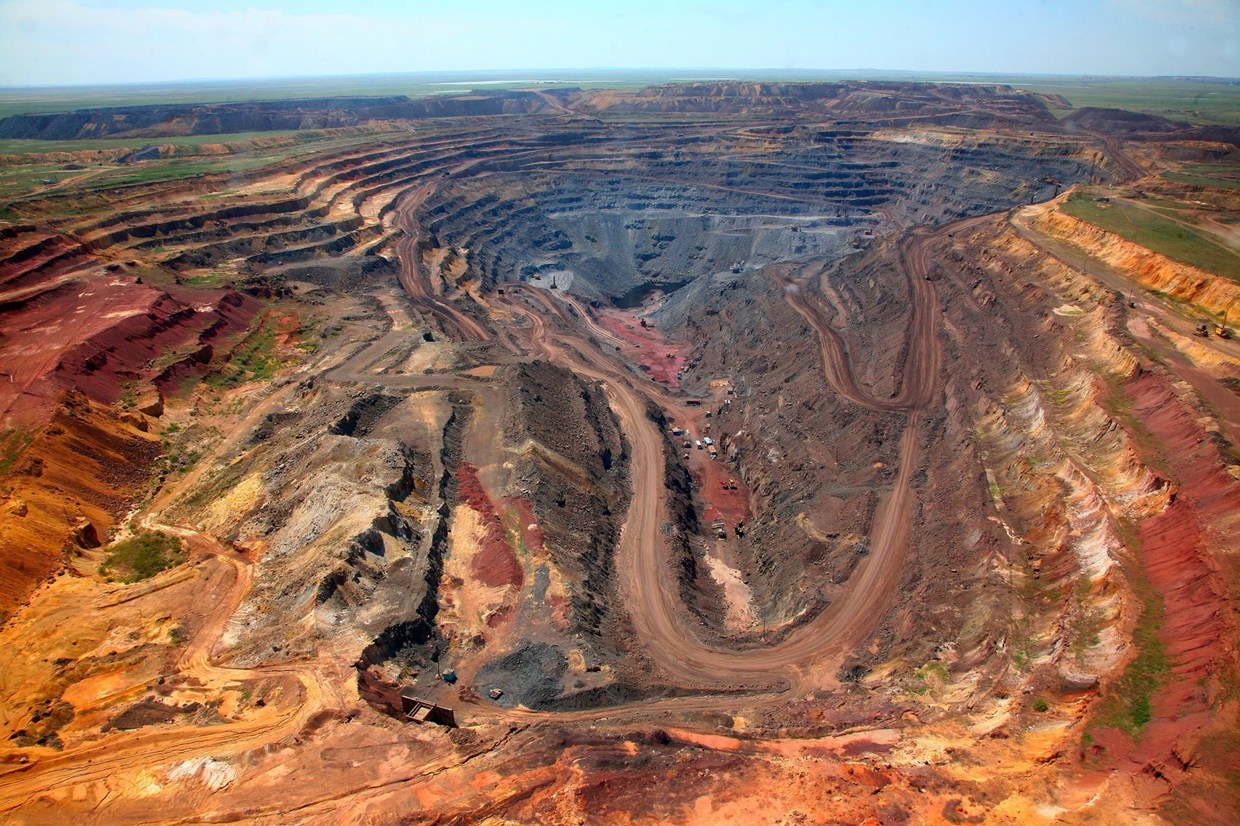
It is widely accepted that low carbon technologies will contribute to reduce greenhouse gas emissions, mostly those coming from carbon dioxide, and thus slow down the global warming. That’s why most of the largest economies in the world have committed to reduce their gas emissions by supporting an unprecedented transition from the current fossil-fuel based economy to the so-called low carbon economy. This new paradigm is considerably less power-consumption intensive and relies on more energy efficient technologies applied, among others, in wind turbines, photovoltaic panels or electric vehicles.
But even if the prospect of less fossil fuel dependent technologies is attractive in order to achieve a more environmentally friendly economy, the materialization of the low carbon economy is not without obstacles, one of the main being that most low carbon technologies rely on scarce metals, including rare earth elements. Lithium, neodymium, tungsten, cobalt, germanium, indium,… all of them are present in new technologies, such as hybrid and electric vehicles, touch screens, mobile phones or solar panels, and all are described as being critical materials.
The term criticality refers not only to scarcity of geological reserves of these elements, but also to many other important factors such as political strategies, commercial barriers, environmental restrictions or limited substitutability of certain materials that can lead to a risk of supply disruption and thus to a sudden failure of indispensable infrastructures and exposure of energy-related activities. It goes without saying that those who own mineral resources hold power, and those who don’t, lose power. And that’s how the low carbon economy starts to sound far less “green”.
The fact that certain mineral deposits are concentrated in very few countries is key to understand the risk of an economy reliant on those minerals. A good example is the domination of China in the production of rare earth elements such as neodymium, an essential component of high-strength magnets used in electronic devices and wind turbines1, or the same kind of monopoly of cobalt in the Democratic Republic of the Congo. Markets dynamics and strategic political decisions could affect essential services when a complete roll-out of the old infrastructures is reached.
That’s why a proper assessment of the criticality of all these elements will be absolutely necessary to face the transition from fossil-fuel to low carbon technologies. Dr Katy Roelich, from the University of Leeds, has focused part of her research on the improvement of the method of stock and flows for the dynamic assessment of material demands in the event of infrastructure transitions2. The result is a model structure in which the stocks and flows of infrastructures, technologies and materials are considered in a dynamic, hierarchical and nested network (figure 2), rather than previous analysis, which were limited to the study of static and disconnected systems. In this way, the model is able to assess, among others, the material dependence of certain technology, component or infrastructure, its lifetime, or the risk mitigation that can be achieved through reuse and recycling.
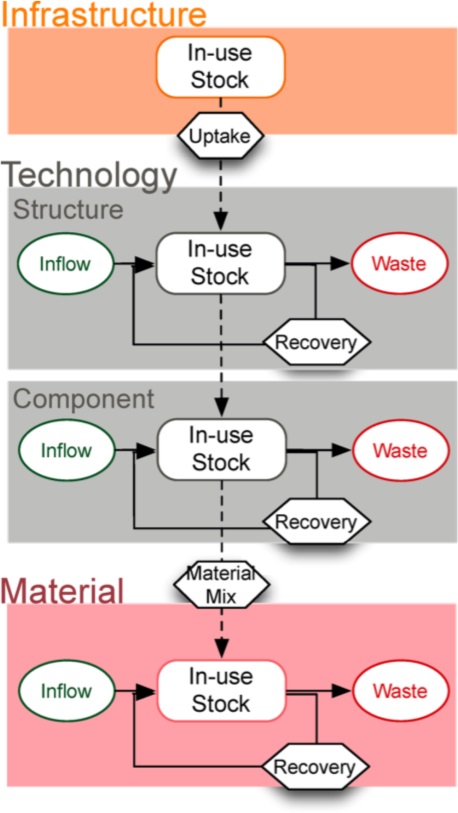
In a later study3, Roelich also proposes a method to analyze material criticality as the combination of two different concepts:
-
Potential for supply disruption, P(t), in which a range of factors are considered, such as constraints on the expansion of production, market imbalances, governmental interventions through regulations, geological scarcity and substitution or recycling of the specified material.
-
Exposure of supply disruption, E(t),which accounts for the impact that the supply disruption of a material can have on a specific system, such as low carbon infrastructure. This variable is quantified mainly through the goal and price sensitivity, that is, the likely effect of disruption over price variations and goals of the system.
While the potential for disruption is a property of the material, the exposure of disruption is a property of a particular system. Both are dynamic and their product, C(t) = P(t)×E(t), gives the dynamic criticality for a particular material in a specific system.
When applied to a particular system, the neodymium criticality in low carbon electricity of the United Kingdom, they found that P(t), the potential for supply disruption of neodymium, is likely to be reduced in the next decades. This reduction is mainly due to two different factors: the low environmental restrictions in China, the main producer, and the consideration of finding new geological reserves in the future. On the contrary, the impact of supply disruption on low carbon electricity systems, E(t), will increase in the future mainly due to the prevalence of low carbon technologies over the old ones, which will remarkably increase our dependence on neodymium. Thus, they conclude that criticality of neodymium will increase in the next decades, endangering indispensable infrastructures.
In the light of these prospects, researchers from different areas of knowledge are proposing solutions to face the criticality of these materials and technologies. Recovery and recycling is a good option to start with, but some important issues should be taken into account, such as the current lack of recovery facilities, optimal treatment processes or even the very same materials to recover, as most of them are currently in use, and it will take some decades for them to become waste materials. Moreover, secondary materials, ie, those coming from recovery treatments have important technical limits4.
Another important aspect to have in mind is the improvement of technology designs and modularity in a way that minimizes the need for scarce materials. Even if this strategy will increase initial costs, these costs will be rewarded in the long term. On the other hand, some claim that relying in just one technology is truly risky, and that a suite of different of technological solutions should be working at the same time. Anyhow, a good understanding of all the factors involved in the development of new products and technologies will be essential to start making the right decisions. Governments play a key role in this infrastructure roll-up, and clear and comprehensive objectives are really needed in this matter or carbon emission targets could be missed.
Finally, this is a great opportunity for geologists to explore new geological reserves and to better understand their dynamic processes and assess their economic viability. Mine production cannot adapt quickly to growing demands, and starting a new mining project can take decades due to the huge investments needed and the environmental issues involved. Dr Andrew Bloodworth, from the British Geological Survey, has drawn attention5 to the horror caused by conflicts over minerals such as coltan, and to our current lust for rare metals due to the increasing needs for energy and digital devices. He emphasizes the impossibility of meeting our future demands for scarce materials6. At this point, it is inevitable to bring up the question whether a “green” future is possible in our ever-growing paradigm, or we all are in a kind of “green” naivety.
References
- Gu, Y., Wu, Y., Chen, B., & Tian, X. Study on the standard system of rare earth metals recycling from the NdFeB materials. World Journal of Environmental Research. 4(2), 41-47 (2014) ↩
- Busch J., Steinberger J.K., Dawson D.A., Purnell P., Roelich K., Managing Critical Materials with a Technology-Specific Stocks and Flows Model. Environmental Science and Technology, 48, 1298−1305 (2014) ↩
- Roelich, K, Dawson, DA, Purnell, P, Knoeri, C, Revell, R, Busch, J and Steinberger, JK Assessing the dynamic material criticality of infrastructure transitions: A case of low carbon electricity. Applied Energy 123, 378-386 (2014) ↩
- Dawkins E., Roelich K., Falk R., Chadwick M., Metals in a Low-Carbon Economy: Resource Scarcity, Climate Change and Business in a Finite World (Policy brief) Stockholm Environmental Institute (2012) ↩
- Bloodworth A., Painful extractions, Nature, 517, 142-143 (2015) ↩
- Bloodworth A., Track flows to manage technology-metal supply, Nature, 505, 19-20 (2014) ↩