Superconductivity and the BCS theory
The band theory of metals has been subjected to experimental tests many times and is now the accepted model of the behaviour of conductors and insulators. But it has to be modified when it comes to superconductivity.
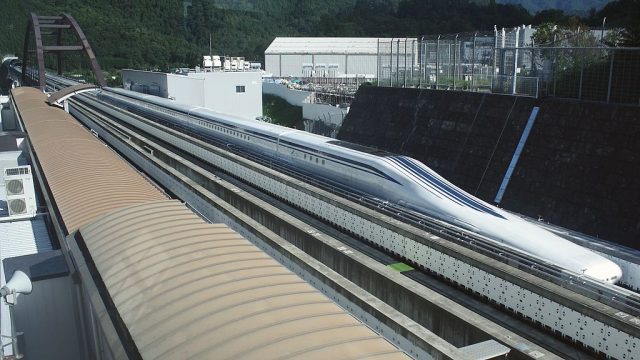
Electrical resistance is due to collisions of the electrons (whether treated as particles or waves) with impurities, imperfections, and especially the lattice vibrations of the metal crystal. The lattice vibrations of the solid will decrease as the temperature falls, because the entropy, which represents disorder, also decreases. Therefore the resistance should also decrease. This is what is observed, and it is well explained by quantum band theory.
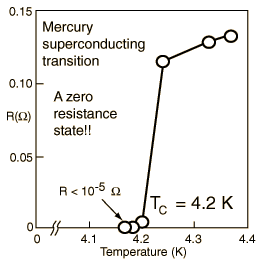
But at very low temperatures something strange happens: the resistance of some materials suddenly drops to zero at a certain temperature. These materials become superconductors, meaning they can conduct currents without resistance or loss of electric energy. The temperature at which superconductivity occurs is called the critical temperature. It ranges from 0.015 K for tungsten to as high as 203 K for hydrogen sulfide (DIPC’s Ion Errea explains how this may be possible here).
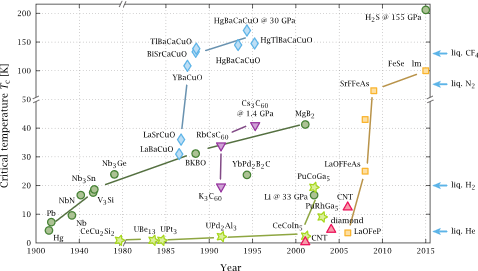
You can imagine some of the possible advantages of electrical wires having zero resistance. It is like motion without friction. Among the many actual and potential applications are electric power transmission lines without power loss, faster computers, and super-strong electromagnets. The latter are used today in magnetic resonance imaging (MRI) devices, high energy particle accelerators, and high-speed levitation trains (often called “mag-lev” for magnetic levitation). Since the boiling temperature of liquid nitrogen, which is plentiful and relatively easy to produce, is 77 K, superconductors with transition temperatures higher than 77 K can be kept sufficiently cold by keeping them immersed in liquid nitrogen. However, such materials are brittle, and the superconductivity can be easily destroyed by stray magnetic fields. Researchers today are working to overcome these problems and to create superconducting materials with transition temperatures even closer to room temperature (20°C, or 293 K).
The development of superconducting devices was greatly stimulated after the acceptance of the basic theory of superconductivity proposed in 1957 by John Bardeen, Leon Cooper, and Robert Schrieffer. The authors of the BCS theory, as it is known, received the Nobel Prize for their work in 1972. Their theory is highly technical but the basic idea is that the electron waves in the superconducting state no longer act independently, as in Bloch’s model. Instead, they are paired together at the critical temperature so that their wave functions act as one unit as they interact with the crystal lattice. Moreover, all of the electron pairs move together in one collective motion, so that if any single electron is scattered by the lattice it is pulled back into the flow by its partner, and if any pair of electrons is somehow scattered off track, it is pulled back into the collective flow by all of the other pairs. Since there is no scattering or inelastic collisions, there is no resistance, and the material becomes a superconductor.
Although the BCS theory accounted well in general for certain crystalline solids, further refinements are required today for other materials, such as ceramics, and for the more detailed behavior of superconductors.
DIPC researchers actively use and investigate superconductivity as the core subject within condensed matter physics that it is. Some of their recent work dealing with superconductors can be found here.
References:
Asimov, I. (1993) New Guide To Science Penguin Press Science
Cassidy, D. et al (2002) Understanding Physics Springer Verlag New York
Nave, C.R. (2012) HyperPhysics
Author: César Tomé López is a science writer and the editor of Mapping Ignorance.
1 comment
[…] por noesbasura | Feb 4, 2016 | Condensed matter, DIPC, Noticias, Physics, Science | 0 […]