Hydrogen gas as alternative fuel
Author: Jelle Vekeman is a Ph.D. student (ITN-EJD-TCCM) at Universitat de València
For a long time now, we have mainly been using fossil fuels to satisfy our increasing needs for energy. Resources, however, are limited and waste products destructive for the environment. As researchers and companies are exploring alternatives, hydrogen gas (H2) seems a promising candidate 1. Unfortunately, adapting our current technologies to this new fuel poses a number of problems, especially concerning storage and transportation of this highly combustible gas 2. The gas needs to be stored at very low temperatures and under high pressures – expensive and possibly dangerous. Storing gas within layers of a solid material, would make transportation less dangerous and therefore cheaper. Hopes are that the adsorption process could offer this possibility 3.
Adsorption is a phenomenon whereby a gas sticks to a surface without entering into the material and without forming chemical bonds. The surface attracts the gas because of small, ever-changing charges within the gas and the surface.
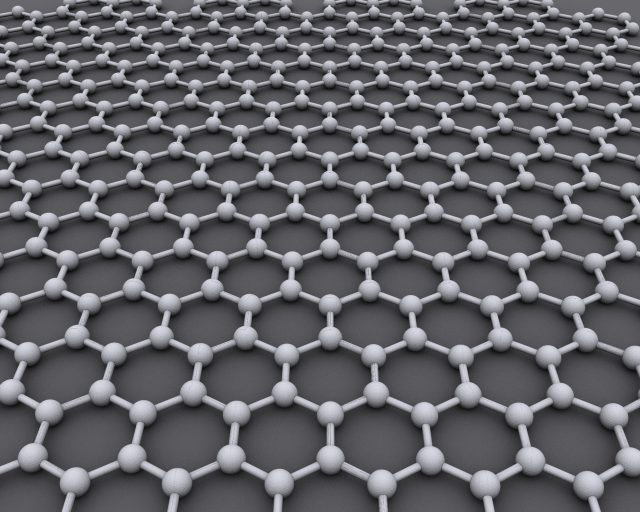
In this work, we will investigate graphene as a possible surface. It is a material composed by carbon atoms arranged in a flat honeycomb structure (see picture 1). Early results have shown this material to be capable of adsorbing interesting amounts of gas. The aim of this work is to characterize this quality over a large temperature range.
First we will study the interaction between the gas molecules and the surface in detail within a small system (7 gas molecules at most) to see how strongly the gas attaches to the surface. We will then use this information to simulate a large amount of molecules in order to get an idea of the temperature range where adsorption is effective.
For the first step, we will make use of density functional theory. The fundamental idea here is that information on a system can be calculated based on its unique electron distribution. Using this technique, we can calculate how strong an interaction between two molecules is. The strength with which a given pair of molecules attract or repel each other depends on the orientation of the molecules and the distance between them.
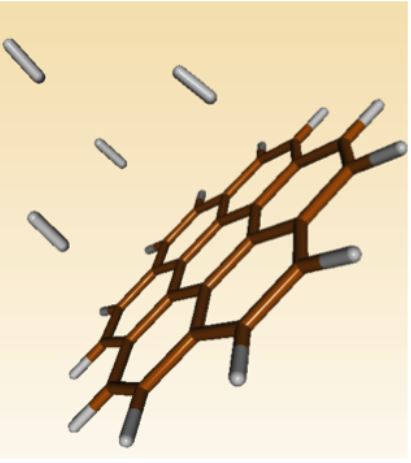
The researchers put an increasing amount of hydrogen molecules (up to 7) in contact with the surface in a fixed orientation at 22 different distances (see figure 2). In figure 3, the interaction energy per H2 molecule is shown for different amounts of molecules in the system. Upon adding a second gas molecule, the interaction energy is lowered significantly. Per extra added molecule hereafter, the energy lowers slowly. In other words, every time an extra gas molecule is added, the already present molecules hinder the new molecule to adsorb to the surface.
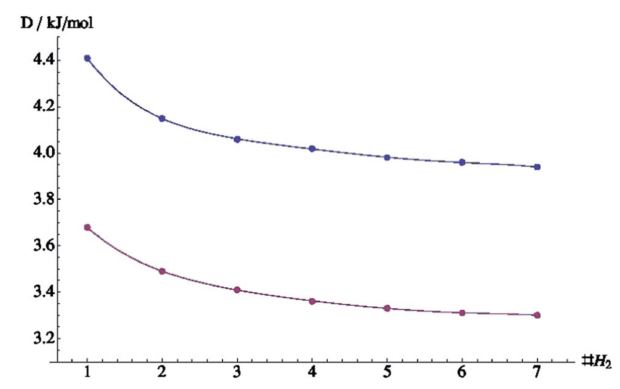
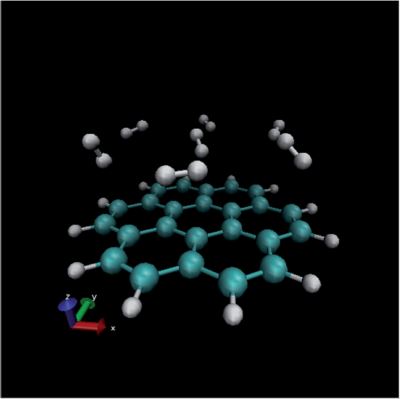
In the previous paragraph, the gas molecules were fixed in orientation relative to the surface. In reality however, gas molecules can take any possible orientation. Therefore, random orientations were generated (see figure 4) and for every one of them the energy was again calculated at 22 different distances. As can be seen from figure 5, the randomly distributed molecules interact less well with the surface (their energies lie higher). This interaction energy will be closer to the real value, since it represents better the real situation within the system.
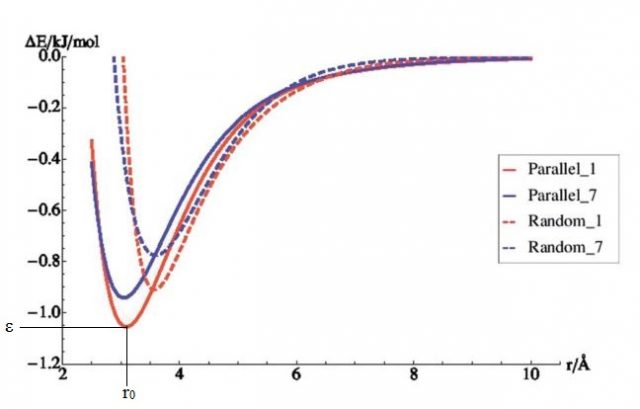
The curves shown in figure 5 have two main features: the equilibrium distance, r0, and the well depth, ε. The first gives the distance with lowest energy; it indicates how far the gas molecule would like to be from the surface. The distance is given in Å, 1 Å being 0.0000000001 meter. ε is the energy value at this specific distance. If the distances become smaller than r0, the energy will rise fast and the system will want to go back to longer distances. If the distance becomes larger than r0, the energy will slowly go to 0 at infinite distance. This actually means that the two systems no longer interact at large distances.
In order to be able to use the plot just discussed, we need a mathematical representation. This can take many forms and in this work, we will use the following 4:
where
Here V(R) is the interaction energy as function of the distance, R. ε and r0 are parameters as explained before, while β (usually taken around 8) is an extra mathematical parameter without physical meaning. The value of m depends on the type of interactions and will be 6 in this case.
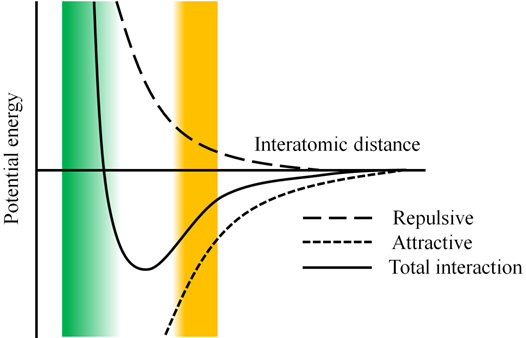
This potential exists out of two parts (see figure 6). The first one (in front of the minus sign) represents the repulsive part of the interaction, the second the attractive. Since the repulsion part has an R-12 dependence, it will go faster to 0 than the attractive part (R-6). Therefore, the outer left of the curve will be determined by the repulsive term, the outer right by the attractive. Finally the middle part of the curve represents the competition between the two interactions.
Now we will search values for ε and r0 so that the mathematical expression represents the curves shown in figure 5 as close as possible. We will do this via a mathematical fitting. The obtained values will be unique for each interaction type, characterizing the behavior of the molecule pair.
Time for the next step in the protocol, the molecular dynamics. In this technique, a lot of gas molecules are brought in contact with the surface. Within our system of gas molecules and the adsorbing graphene, two types of interactions will be important: interactions between the gas molecules themselves and interactions between gas and surface. Special software will check for every gas unit, which molecules it is surrounded by and how they interact. It will then adjust the position of the molecule accordingly. By repeating this process a number of times, a small movie is made showing how the molecules move throughout the system.
The described process was repeated at different temperatures to assess the stability of the adsorption process under different conditions. It was found that for the temperature range of 10K (-263°C) to 390K (117°C), the adsorption energy stays quite constant. This means that the process is very stable within a large temperature range. In other words, the technique should be usable at high and low temperatures.
It was shown, however, that at temperatures above 250K (-20°C), only 4 molecules can stay adsorbed instead of 7. Apparently, the process becomes less effective at higher temperatures in the sense that less molecules can be adsorbed. It should be taken into account that only a very small part of graphene was considered here. When extending to a large portion of graphene, significant amounts of gas should become usable for safer transport. Evidently a lot of follow-up research is needed to further characterize the precise process under different conditions.
References
- Md Bin Yeamin, N. Faginas-Lago, M. Albertí, I. G. Cuesta, J. Sánchez-Marín and A. M. J. Sánchez de Merás, RSC Adv., 2014,4, 54447-54453 ↩
- M. Bartolomei, E. Carmona-Novillo, G. Giorgi, Carbon, 2015, 95, 1076-1081 ↩
- Qinyu Wang and J. Karl Johnson, The Journal of Chemical Physics, 1999,1 110, 577 ↩
- F. Vecchiocattivi, F. Pirani, P. Casavecchia and D. Cappelletti, Phys.Chem. Chem.Phys., 2008, 10, 5489–5503 ↩
4 comments
Nice article Jelle,
…so then, can we avoid the need of low temperature and high pressure associated with hydrogen gas transport when the gas is adsorbed in graphene? Do you have any estimate as to whether the amount of hydrogen which can be potentially transported is meaningful in terms of cost? This is quite an interesting subject, indeed. Thanks.
Dear Sergio
First of all thank you! To answer your question: it is indeed the idea that high pressures and low temperatures would not be necessary during transportation. Concerning your second question, I can say this: The US department of energy has set a target for storage to be useful for commercial use (9.0 wt% gravimetric density and 81 g L−1 volumetric capacity [1]). This target has not been reached yet at ambient conditions to the best of my knowledge. At this moment, research is pointed towards identifying the right materials (graphene is not the only promising candidate) and circumstances for adsorption. So, no I don’t have a direct estimate at present.
[1] D.-E. Jiang, Z. C. (Eds)., Graphene Chemistry: Theoretical Perspec- tives, Springer-Verlag, John Wiley & Sons, 2013.
Quite useful info, indeed. So there are other options available…promising area of research/development with huge industrial and financial implications. Keep us updated if any new progress arises.
Cheers,
S.
[…] Si pretendemos que alhuna vez el hidrógeno sea un combustible alternativo a la gasolina, se bedería poder transportar y manejar con la seguridad que ahora mismo tiene ésta. ¿Por qué no usar grafeno para conseguirlo? Jelle Vekeman en Hydrogen […]