Ab initio modeling the chemical storage of alternative energy
Author: Carles Martí is a Ph.D. student (ITN-EJD-TCCM) at University of Perugia
Nowadays, mostly everybody has heard about global warming, a problem that humanity faces and that can drastically change our climate in the upcoming years. This is caused by the, also well known, greenhouse effect, produced by the gases our modern society generates, to keep our life standards. The main one is carbon dioxide (CO2), generated when fossil fuels are burnt for multiple purposes: producing electrical energy in thermal power plants, making our transport vehicles move, or generating heat for industrial processes. Another inconvenient fossil fuels have is their limited availability. The fact that energy consumption demand increases exponentially while their reserves are getting depleted makes the current energy model to have a near expiry date.
In order to deal with these two problems, many solutions were proposed over the years. One of the first ones was the discovery of the biofuels. They were thought to be the future: with a minimum change in the energy model and facilities, society could do a fairly simple change into a renewable energy source, enough to supply a large part of the transport that industry needs. However, their problems came in 2007 when the price of corn exponentially grew due to its renewable boom. This had dramatic effects in the economy of Mexico and part of Latin America. In fact, families saw how their main alimentary product, the corn made tortillas, became unaffordable in the so called “Tortilla crisis”. From that moment, another requirement was added to the energy sources to become a valid one: it shouldn’t interfere with the food industry.
Given this new requirement, one of the most environmentally friendly solutions is the use of other renewable energy sources like photovoltaic, eolic, hydraulic, waste biomass, etc… However they all have a problem, their energy production is not constant over time. Photovoltaics production depends on the weather: apart from the difference in day-night production, on sunny days more electricity is produced than on cloudy ones. Something similar happens with other natural energy sources, eolic depends on the wind, hydraulic on the season-dependent rain, etc as can be seen in Figure 11.
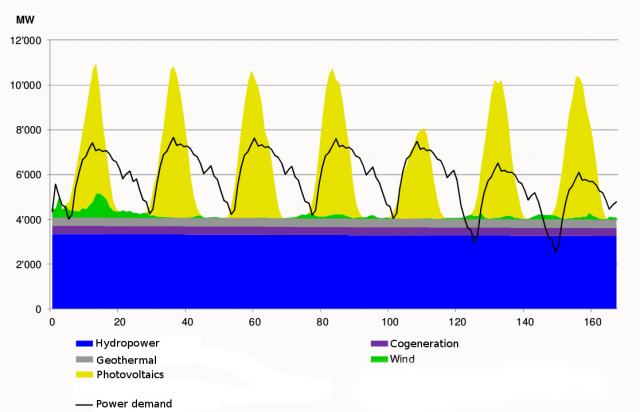
Therefore, a way of storing energy is needed. Furthermore, it has to be efficient, easy and fast to reconvert it back to electrical power and, if possible, versatile. The goal is to take the energy in excess when a peak of production occurs, store it, and use it whenever (and if possible wherever) needed. In that sense the Sabatier reaction, combined with the electrolysis of water, can deal with all the outlined problems. The storing procedure consists on, firstly, do the electrolysis of water with the excess electricity produced, to obtain hydrogen. Secondly, make this hydrogen react with carbon dioxide (this way we get rid of the main responsible gas producing the greenhouse effect), to produce carbon neutral methane and water. With that procedure we succeed to produce an energy vector, methane, which:
-
Is easily manageable with today’s facilities (we are actually talking of natural gas).
-
Has a high energetic density (the highest of all hydrocarbons).
-
Is Carbon neutral, ie. no neat CO2 is produced when burnt (since it’s produced by the recycling of carbon dioxide).
-
Is versatile, it can be reconverted into electrical power in thermal power plants, used as fuel for vehicles, or consumed at houses like it’s nowadays done (to heat water, cook, etc.).
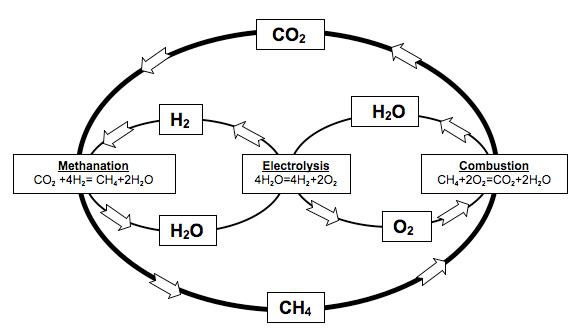
Research in that field is, thus, a must for today’s society. From this point of view, theoretical chemistry can play a key role. The role of explaining the detailed mechanism of how the reaction occurs, which can help in developing new catalysts or reaction conditions. The first step is the understanding of the kinetics of the Sabatier reaction. In spite of being a quite simple reaction: CO2 + H2 → CH4 +H2O, its kinetics is far more complicated. The reaction, instead of happening in one single step proceeds via a sequence of little (elementary) steps of which the overall sum is also called mechanism of reaction 2. Firstly, the reactants (H2 + CO2) become intermediates. And they react between each others in a large list of elementary reactions to finally become products (CH4 + H2O). As much is the complication of this mechanism that there is yet no consensus in scientific community on which is the exact elementary reactions (or steps) and its intermediate species.
Furthermore, every elementary step doesn’t contribute with the same weight to the overall reaction velocity. The slower ones are more important than the faster ones, a way of understanding this fact would be to think on a 5-lane highway which in a 10 km sector has workings on it and only two are available. The overall velocity is conditioned by the 10 km sector more than on the 5 lane one. Same happens with reactions, if a mechanism of reaction is composed, to simplify, of 2 successive steps, let’s say the second is very fast (it lasts only 0,1 s) while the first is very slow (it takes 100 s to complete). Even if the second step is very fast, it has to wait the first to end, since it needs its products to start the reaction, it is clear then, that the overall reaction time would be the sum of the times of every step (ie. 100+0,1=100,1) which is practically the same as the velocity of first step. One can extend this fact to a more complex mechanism with multiple steps.
From this fact it is clear the importance of knowing how a reaction mechanism occurs and which are the velocity of its steps. For this purpose, we have carried out calculations using two methods, kinetic Monte Carlo to simulate the overall mechanism velocity 3 and Quantum reactive dynamics to have an exact view of the most important elementary steps 4.
References
- Dr. Almut Kirchner, Daniel Bredow, Florian Ess, Dr. habil. Thomas Grebel, Peter Hofer, Dr. Andreas Kemmler, Andrea Ley, Dr. Alexander Piégsa, Nadja Schütz, Samuel Strassburg, Jutta Struwe, Mario Keller (Infras AG), Die Energieperspektiven für die Schweiz bis 2050, Bundesamt für Energie (BFE), (2012) ↩
- Aziz, M. A. A., Jalil, A. A., Triwahyono, S., & Ahmad, A. Green Chem., 17 (5), 2647-2663. (2015) doi: 10.1039/C5GC00119F ↩
- Martí, C., Pacifici, L., Capriccioli, A., & Laganà, A. Computational Science and Its Applications – ICCSA 2016. Proceedings, Part I, 319–333 (2016).doi:10.1007/978-3-319-42085-1_25 ↩
- Martí Aliod, C., VIRT&L-COMM. 9.2016.5 (2016). ↩
1 comment
[…] Hay formas y formas de estudiar cómo almacenar las cosas. Una es ponerte a probar y otra, muy diferente, es empezar por el principio. Carles Martí en Ab initio modeling the chemical storage of alternative energy. […]